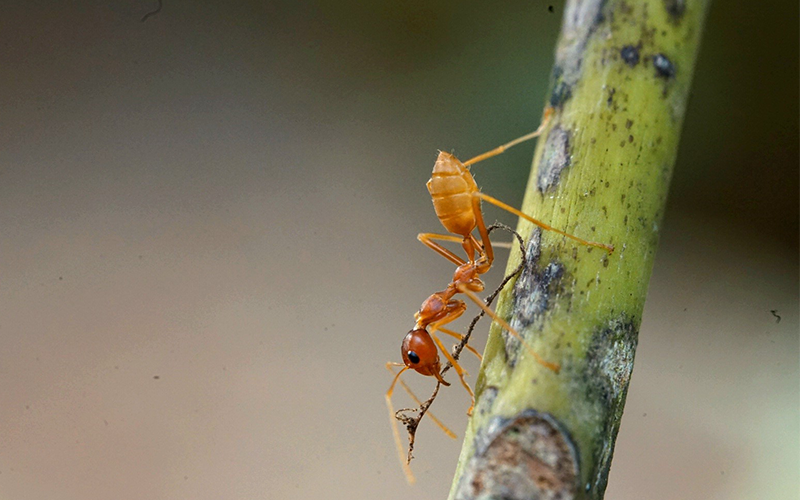
Footprints in the agar: growing bacteria from ants’ feet to combat plant diseases
A walk on the wild side: invite some ants to take a walk on your petri dish and discover how bacteria from their feet could help us reduce pesticide use.
Talking trees: discover how trees use chemicals to communicate with soil microbes and how this could impact their ability to act as carbon sinks.
Like humans, trees require various elements to thrive. But with many of those elements locked away in complex soil molecules, how do they access what they need? Trees have developed their own language with soil microbes to trade carbon fixed by photosynthesis for nutrients like bioavailable nitrogen. This dialogue not only sustains tree growth but also holds significant implications for climate change mitigation. Forests serve as carbon sinks and their capacity to absorb carbon dioxide (CO2) relies on nutrient availability, thus underscoring the pivotal role of this microbial language in our planet’s future.
Trees, much like humans, need to be supplied with a diverse mix of nutrients to keep growing. These nutrients contain chemical elements that plants need for growth. More or less the same elements are required for both humans and plants to build and maintain genetic code, proteins, cell walls, etc. The most important elements include carbon (C), nitrogen (N), phosphorus (P), oxygen (O), potassium (K), magnesium (Mg), and calcium (Ca). However, unlike humans who can actively seek out these nutrients in their environment, trees are anchored in place and rely on the reach of their roots and symbiotic fungal mycorrhizae to scavenge for nutrients. But are all these elements present in the soil? Yes and no. Yes, they are all present but usually not in a form available for plant uptake (i.e. not edible). All these elements are usually found in organic and mineral molecules (Figure 1), but these molecules are too complex and too large to be absorbed by root cells. This is where soil microbes come in! Soil bacteria and fungi possess a unique ability to produce enzymes capable of breaking down these complex molecules into smaller, more accessible forms, such as sugars, amino acids, and inorganic nitrogen (N) compounds like ammonium (NH4+) and nitrate (NO3–), which can be directly utilized by trees. As microbes break down these molecules, they absorb a portion of the released nutrients to integrate in their cells and grow in numbers and release leftover molecules into the soil. This leftover supply of nutrients can then be taken up by trees to integrate into their own cells. This dynamic is particularly critical for nitrogen as it frequently limits tree growth in northern hemisphere forests.
The ground-breaking invention of the Haber–Bosch process in the early 20th century revolutionized agriculture by efficiently fixing plentiful but inert atmospheric nitrogen into compounds that plants can use, such as ammonium salts with nitrogen in the form of ammonium ions (NH4+). This innovation facilitated rapid food production, catalyzing agricultural growth worldwide. However, the excessive use of nitrogen fertilizer over subsequent decades is now considered a significant contributor to climate change[2,3] and has led to a non-uniform distribution of fixed nitrogen across the globe. Regions near industrial and agricultural areas experience detrimental effects from excess nitrogen fertilizers, such as high emissions of nitrous oxide – a potent greenhouse gas – and the contamination of water bodies with nitrates. Conversely, ecosystems situated far from industrial and agricultural zones, like some forested areas, continue to face a scarcity of nitrogen compounds that plants can use, which constrains their productivity and resilience.[4]
As our planet heats up due to human activities releasing CO2 into the air, trees may play a crucial role in absorbing this excess CO2 through photosynthesis.[5] Their ability to act as carbon sinks makes them powerful allies in slowing down climate change, but trees can only absorb excess CO2 if they are supplied with enough nitrogen. In forests where soil nitrogen is scarce, studying nitrogen availability in soils is complex; it’s like resolving multiple equations with multiple unknowns, all of them dependent on soil microbes. Nitrogen availability in soils is regulated by the nitrogen cycle, defined as the repeating processes by which nitrogen cycles between inorganic and organic forms, and moves between biotic (living) and abiotic (non-living) systems, including the atmosphere, soil, water, plants, animals, and bacteria (Figure 2). In fact, plant growth is often limited by nitrogen mineralization (the breakdown of complex organic compounds into mineral nutrients), greenhouse gas emissions are a function of denitrification (the production of nitrogen gas from nitrate), and groundwater pollution is a result of nitrate leaching (loss of nitrate from the upper soil). The speeds of these processes are dependent on climatic parameters, such as soil moisture, temperature, and the availability of other nutrients. Consequently, these processes, and by extension nitrogen availability, are likely to be influenced by climate change. Think of it like a seesaw – if it gets unbalanced, too much nitrogen can turn into greenhouse gases or be leached into groundwater, leaving insufficient nitrogen in the soil for trees to thrive.
Scientists around the world study nitrogen availability in forests in experiments designed to model expected conditions to understand how forests can help mitigate climate change. To do so, researchers use free-air CO2 enrichment (FACE) experiments, which mimic the predicted concentration of atmospheric CO2 in 2050 by using tall vertical pipes blowing CO2 in areas of mature forests (Figure 3). In these experiments, researchers study tree growth, analyze root exudate composition, assess microbial activity, and use isotopic tracing techniques to measure nitrogen availability in soils.
Researchers at the University of Birmingham, UK, are finding that, when available soil nitrogen is limited, trees can trade the one element it can access more easily than microbes – carbon – for nitrogen. Trees fix carbon via photosynthesis in their leaves and convert it into glucose, so they can easily redistribute part of this to microbes in the soil through root exudates, which are fluids released through plant roots and are usually rich in carbon. This dynamic is amplified under elevated atmospheric CO2 concentrations because trees use the excess CO2 to fix more carbon. Extra carbon released into the soil via root exudation stimulates microbial activity, which accelerates the decomposition of soil organic matter and leads to the release of nitrogen in a form trees can use (Figure 2).[6] But this partnership between microbes and plants goes beyond simple food exchange. Indeed, trees not only release simple glucose; they can selectively adjust the composition and concentration of the compounds released into the soil to enhance or inhibit specific microbial functions. For example, under conditions of high atmospheric CO2, trees may release compounds to inhibit nitrification, like ferulic acid,[7] to prevent nitrogen loss from the soil. By doing so, trees maintain nitrogen in their preferred form, ammonium, and reduce potential losses to the atmosphere and groundwater. Thus, the composition and concentration of these exudates act like an encoded message, telling microorganisms what is needed for optimal plant growth. However, grasping the intricacies of this communication remains a challenge. Continued efforts are underway to translate this plant-to-microbe language, since it could hold a key to understanding how forests can help us mitigate climate change.
[1] Schulten HR, Schnitzer M (1997) Chemical Model Structures for Soil Organic Matter and Soils. Soil Science 162: 115–130.
[2] Steffen W et al. (2015) Planetary boundaries: Guiding human development on a changing planet. Science 347: 1259855–1259855. doi: 10.1126/science.1259855
[3] Galloway JN, Cowling EB (2021) Reflections on 200 years of Nitrogen, 20 years later. Ambio 50: 745–749 doi: 10.1007/s13280-020-01464-z
[4] Townsend AR, Howarth RW (2010) Fixing the global nitrogen problem. Scientific American 302: 64–71. doi: 10.1038/scientificamerican0210-64
[5] Gardner A et al. (2021) Is photosynthetic enhancement sustained through three years of elevated CO2 exposure in 175-year-old Quercus robur? Tree Physiology 42: 130–144. doi: 10.1093/treephys/tpab090
[6] Dijkstra FA et al. (2013) Rhizosphere priming: a nutrient perspective. Frontiers in Microbiology 4. doi: 10.3389/fmicb.2013.00216
[7] Nardi P et al. (2020) Biological nitrification inhibition in the rhizosphere: determining interactions and impact on microbially mediated processes and potential applications. FEMS Microbiology Reviews 44: 874–908. doi: 10.1093/femsre/fuaa037
The article addresses an important dimension of the biosphere’s capacity to face the challenges posed by the increasing of greenhouse gases: interactions between species from different kingdoms of life, specifically, trees and soil microorganisms, in a dynamic engaged around the essential nutrient nitrogen.
Science teachers can use this text to promote a better understanding of the nitrogen cycle in nature, as well as to stimulate interest in the topic of climate change through questions such as:
Luis M. Aires, science teacher, Antonio Gedeao School Cluster, Almada, Portugal
A walk on the wild side: invite some ants to take a walk on your petri dish and discover how bacteria from their feet could help us reduce pesticide use.
Trees are incredible chemical factories that capture carbon dioxide and produce the oxygen we breathe, but they produce other chemicals too. We need…
Biology, maths, and the SDGs: estimate the CO2 absorbed by a tree in the schoolyard and compare it to the CO2 emissions of a short-haul flight.