How trees affect the climate: is it just through photosynthesis? Understand article
Trees are incredible chemical factories that capture carbon dioxide and produce the oxygen we breathe, but they produce other chemicals too. We need to understand their effect in the atmosphere to get the most out of tree-planting initiatives.
Climate change and air quality are pressing concerns in the 21st century. Both are linked to the burning of fossil or biofuels, which produces carbon dioxide, sulphur dioxide, nitrogen oxides, and a variety of particulates. Poor air quality is known to be bad for human, plant, and animal health, which will also be affected by climate change. Predictions of the global average temperature and rainfall depend on an accurate understanding of airborne compounds and how their concentrations are likely to change as populations increase and policy and temperature changes.
Global tree planting
Carbon dioxide (CO2) is the main greenhouse gas responsible for trapping heat in the atmosphere. One way to offset climate change is through global tree planting. [1] Trees convert CO2 into glucose through photosynthesis (equation 1).
When glucose is turned into cellulose, the CO2 becomes trapped until the wood is burnt or rots. Immediate action to replant approximately 0.9 billion hectares (Ha) of forest could remove (sequester) up to 200 gigatonnes of CO2. [1] This is equivalent to ~25% of the current global atmospheric carbon load. Research has shown that this would be most effective in the tropics.
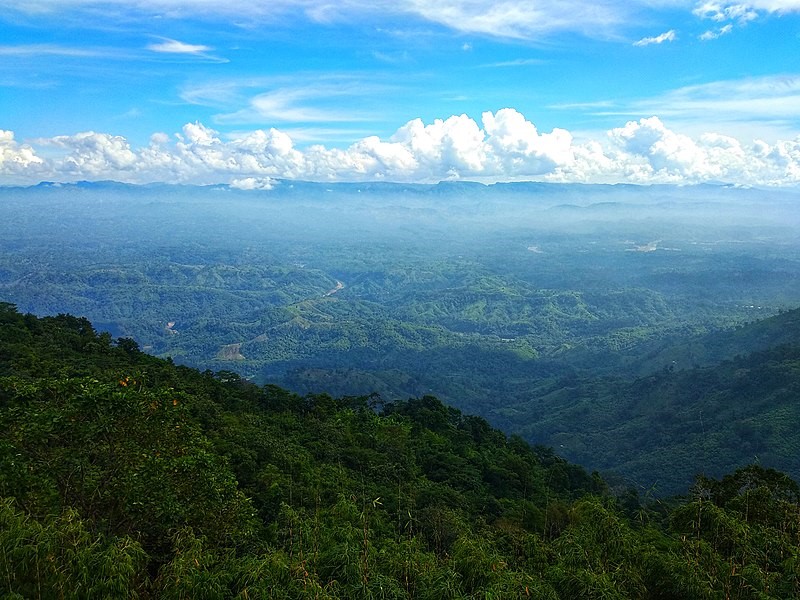
Image: Madhaabiii/Wikimedia, CC BY-SA 4.0
However, photosynthesis to remove CO2 is not the only way in which trees affect the earth’s atmosphere. Trees can provide cooling and shading during summer months and provide shelter from winds and rain during winter months. Tree roots and increased levels of soil organic matter from leaf litter inputs improve soil structure, enhance aggregate stability, and promote fungal activity, leading to higher porosity. In addition, tree produce and release a number of different compounds to support their own life processes. It is these compounds that give pine forests their scent, for example. In the presence of pollutants such as NOx, some of these compounds, including isoprene and terpenes, can act as biogenic volatile organic compounds (BVOCs). These chemicals can react with oxidants in the atmosphere [e.g., hydroxyl radical (•OH), ozone (O3), nitrate radical (NO3•)] to create secondary pollutants, such as more ozone (O3), a short-lived greenhouse gas, which that is also known to can reduce crop yields, and secondary organic aerosols (SOAs) that can have significant impact on climate. These secondary pollutants are short-lived but also affect the Earth’s ability to reflect heat energy back into space. The potential short- and long-term impact of these compounds on climate change on habitats, food security, and land use are of great concern for urban tree planting if NOx emissions are not also controlled.
Key terms
Term | Meaning |
---|---|
Aerosol | A suspension of fine solid particles (particulates) or liquid droplets in a gas or air. |
Biogenic volatile organic compounds (BVOCs) | Naturally formed carbon-based molecules that easily form vapours and can react with other compounds in the atmosphere. |
Greenhouse gases | Gases, such as carbon dioxide, methane, water vapour, and nitrous oxide (N2O), gases that trap infrared radiation in the atmosphere and lead to warming. |
Hydroxyl free radical (HO•) | A highly reactive species containing an unpaired electron and formed in the atmosphere. It attacks most organic molecules. |
NOx | A highly polluting mixture of oxides of nitrogen, mainly nitrogen dioxide (NO2) and nitrogen oxide (NO) gases formed by combustion at high temperature. |
Ozone (O3) | A toxic form (allotrope) of oxygen. In the upper atmosphere the ozone layer limits incoming UV radiation reaching the surface of the Earth. However, in the lower atmosphere (the troposphere), ozone forms in a series of reactions between NOx and VOCs and is a greenhouse gas. |
Secondary organic aerosols (SOAs) | Chemicals produced after several generations of oxidation of a parent organic molecule. |
Secondary pollutants | Pollutants formed through reactions with primary pollutants. |
Terpenes | A class of (predominantly) plant compounds with the formula (C5H8)n comprising more than 30 000 compounds |
Teratonne | A trillion tonnes |
There are many key factors to consider when initiating a world-wide tree restoration program. We need to understand the chemicals that trees produce at different temperatures and how their breakdown products affect climate and air quality. For example, urban tree planting projects are promoted to remove particulates and provide green spaces for improved human well-being. But in the presence of elevated concentrations of NOx gases (e.g., from traffic), the release of BVOCs by trees can accelerate the formation of tropospheric O3 and negatively impact air quality and climate. [2] The overall impact of urban tree planting may not necessarily be negative because trees still fulfil important functions in urban areas, but it is necessary to take this additional impact of NOx pollution into account and control NOx emissions.
Key BVOCs
Isoprene
Isoprene is a plant-derived unsaturated hydrocarbon with a global emission of >500 teratonnes per year. [3] At very low NOx concentrations (less than 50 parts per trillion) isoprene can be helpful because its degradation products can catalyse the formation of HO., an atmospheric ‘chemical detergent’ that accelerates the removal of organic pollutants and reduces regional greenhouse gases such as methane (CH4).[4–7] However, if there are high NOx concentrations in the areas around the trees, isoprene can lead to the formation of secondary pollutants.
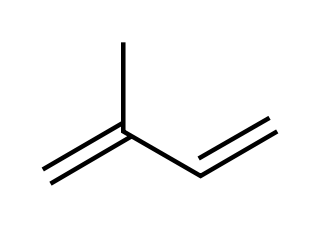
Terpenes
These chemicals, mainly derived from plants, consist of monoterpenes and sesquiterpenes with estimated emissions of around 150 and 30 teratonnes per year, respectively.[3] While isoprene is only emitted by trees during daylight, terpenes are emitted throughout the day. These unsaturated, highly reactive hydrocarbons have many functions in plants, including attracting pollinators, wound healing, attracting organisms to remove predators, communication, ripening, protection from UV radiation, and removal of reactive oxygen species, notably O3, through fast reactions.[8,9] Terpenes often accumulate in specialized structures like resin ducts[9] from where they evaporate and react rapidly, with lifetimes of minutes to hours depending on the structure, location, and oxidant level. There is often still residual terpene present in dead plant material, which makes forest litter more combustible. Therefore, terpenes also play a role in accelerating forest fires. On the other hand, terpenes can be beneficial by altering tropospheric chemical composition and leading to cooling of the environment through SOA formation providing cloud seeding nuclei. [10]
Monoterpene | Common Sources | Examples of Use |
---|---|---|
Myrcene | Wild thyme, hops, cannabis, cardamom | Precursor for several fragrance molecules |
α-Pinene | European pine trees, rosemary, cannabis, eucalyptus, orange peel oil | Solvent, insect repellent, precursor for some fragrances |
Sabinene | Marjoram, Norwegian Spruce, Holm Oak | Skin inflammation and dermatophytosis treatment; antibacterial agent effective against gram-positive bacteria |
Limonene | Found in citrus fruit oils | Solvent, food flavouring, fragrances, insecticide |
Camphene | Found in many essential oils | Fragrance precursors and food additive for flavouring |
Terpenes are not only key chemical species in tropical environments. They also have influence across the whole Earth. In summer of the boreal forests of the northern hemisphere, where perpetual daylight occurs, terpenes play a critical role in modifying the climate through aerosol formation. This may alter the radiative balance in these regions by changing from local atmospheric cooling to a warming scenario. Five groups of tree-produced monoterpenes have been defined (figure 1) based on key carbocation intermediates in the chemical reactions that form them. As the temperature changes, monoterpene emissions change from one group to another as different biochemical pathways are switched on or off in response to the conditions.
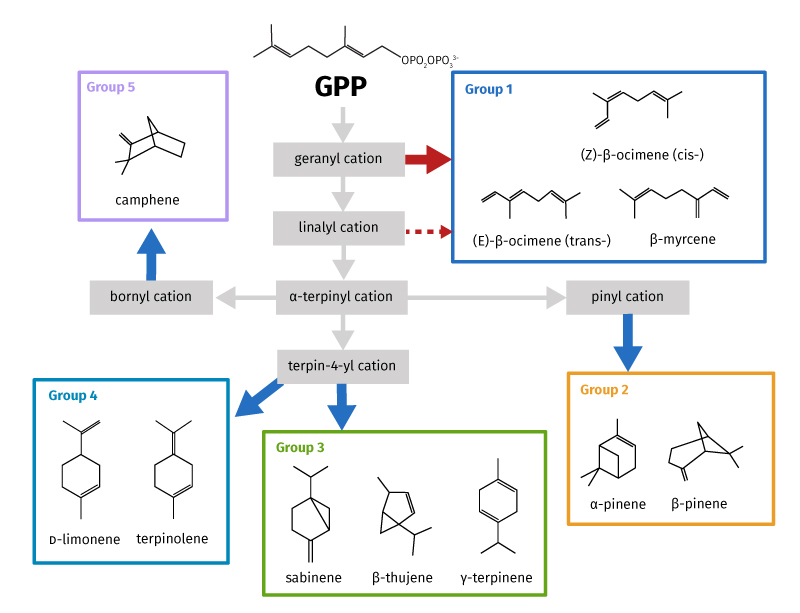
Image: Christina Hof/Science in School, CC-BY
Recent research in the Amazon showed that as leaf temperature rose from 27 to 35°C, Group 4 and Group 2 monoterpenes declined as the main species present (from ~80% to 45%) whereas Group 1 rose from ~10% to 50%. [11]
Why is this important? In most atmospheric chemistry models used to predict climate change, the impact of just one or two monoterpenes from Group 2 are usually considered, as they were thought to be the dominant species. This has now been shown not to be the case at increased temperatures.
The current challenges
Understanding the mechanisms by which these compounds break down is challenging, and our understanding is far from complete. A more complete mechanism for the breakdown of BVOC molecules shows that second, third, and possibly fourth generation breakdown products can have impacts on climate: cooling may be much more important than first estimated. [12] Knowing what the breakdown products in each step are, and how long they will last in the atmosphere, will allow more accurate calculations of the effect on the climate. It is thought that as temperature increases, Group 1 emissions start to dominate so their breakdown products should be more thoroughly researched; currently low-temperature Group 2 compounds are used in climate model calculations. Although monoterpenes react rapidly with key oxidants in the atmosphere, the lifetimes of different species differ significantly. Thus, their role in shaping local to regional, and even global, chemical compositions will vary dramatically. For example, in clean conditions (very low NOx concentrations) found in tropical forests, the lifetime of Group 1 terpenes is much shorter than all other classes. [13–17] This reduces the distance over which the impact of breakdown products may occur.
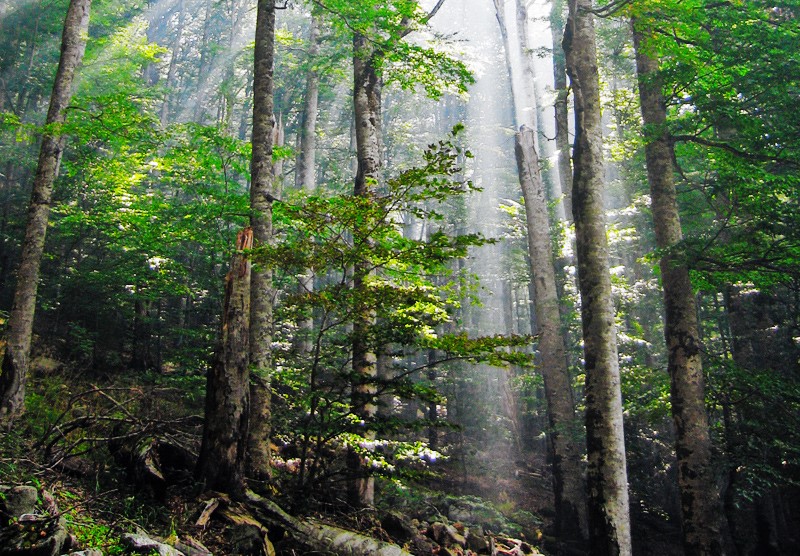
Snežana Trifunović/Wikipedia, CC BY-SA 3.0
It is not just planting more trees that is important, but planting the right species at the right latitude. Failing to restore forests or, far worse, diminishing them further, will have an impact beyond increasing CO2 levels. Deforestation will lead to rising surface temperatures that will increase the emissions of such chemicals. A key barrier to our understanding of the climate impacts of tree planting is a lack of knowledge of the chemical breakdown mechanisms for the five groups of terpenes. This information could be fed into predictive climate change models to improve their accuracy.
References
[1] Bastin JF et al. (2019) The global tree restoration potential. Science 365: 76–79. doi: 10.1126/science.aax0848
[2] Zhao DF et al. (2017) Environmental conditions regulate the impact of plants on cloud formation Nature Communications 20: 1–8. doi: 10.1038/ncomms14067
[3] Guenther AB et al. (2012) The Model of Emissions of Gases and Aerosols from Nature version 2.1 (MEGAN2.1): an extended and updated framework for modeling biogenic emissions Geoscientific Model Development 5: 1471–1492. doi: 10.5194/gmd-5-1471-2012
[4] Bates KH, Jacob DJ (2019) A new model mechanism for atmospheric oxidation of isoprene: global effects on oxidants, nitrogen oxides, organic products, and secondary organic aerosol Atmospheric Chemistry and Physics 19: 9613–9640. doi: 10.5194/acp-19-9613-2019
[5] Jenkin ME et al. (2019) The CRI v2.2 reduced degradation scheme for isoprene Atmospheric Environment 212: 172–182. doi: 10.1016/j.atmosenv.2019.05.055
[6] Lelieveld, Jet al. (2008) Atmospheric oxidation capacity sustained by a tropical forest Nature 452: 737–740. doi: 10.1038/nature06870
[7] Muller JF, Stavrakou T, Peeters J (2019) Chemistry and deposition in the model of atmospheric composition at global and regional scales using inversion Techniques for trace gas emissions (MAGRITTE v1.1.)-Part 1: chemical mechanism Geoscientific Model Development 12: 2307–2356. doi: 10.5194/gmd-12-2307-2019
[8] Pinto DM et al. (2010) Plant volatile organic compounds (VOCs) in ozone (O3) polluted atmospheres: the ecological effects Journal of Chemical Ecology 36: 22–34. doi: 10.1007/s10886-009-9732-3
[9] Holopainen JK (2011) Can forest trees compensate for stress-generated growth losses by induced production of volatile compounds? Tree Physiology 31: 1356–1377. doi: 10.1093/treephys/tpr111
[10] Ranero Celius K (2010) Clouds: puzzling pieces of climate Science in School 17: 54–59.
[11] Jardine KJ et al. (2016) Monoterpene ‘thermometer’ of tropical forest-atmosphere response to climate warming Plant, Cell & Environment 40: 441–452. doi: 10.1111/pce.12879
[12] Khan MAH et al. (2017) A modeling study of secondary organic aerosol formation from sesquiterpenes using the STOCHEM global chemistry and transport model JGR Atmospheres 122: 4426–4439. doi: 10.1002/2016JD026415
[13] Atkinson R, Aschmann SM, Pitts JN Jr. (1986) Rate constants for the gas-phase reactions of the OH radical with a series of monoterpenes at 294 ± 1 K International Journal of Chemical Kinetics 18: 287–299. doi: 10.1002/kin.550180303
[14] Atkinson R, Aschmann SM, Arey J (1990) Rate constants for the gas-phase reactions of OH and NO3 radicals and O3 with sabinene and camphene at 296±2 K Atmospheric Environment 24: 2647–2654. doi: 10.1016/0960-1686(90)90144-C
[15] Gill KJ, Hites RA (2002) Rate Constants for the Gas-Phase Reactions of the Hydroxyl Radical with Isoprene, α- and β-Pinene, and Limonene as a Function of Temperature Journal of Physical Chemistry A 106: 2538–2544. doi: 10.1021/jp013532q
[16] Atkinson R, Hasegawa D, Aschmann SM (1990) Rate constants for the gas-phase reactions of O3 with a series of monoterpenes and related compounds at 296 ± 2 K International Journal of Chemical Kinetics 22: 871–887. doi: 10.1002/kin.550220807
[17] Atkinson R, Winer AM, Pitts JN Jr. (1982) Rate constants for the gas phase reactions of O3 with the natural hydrocarbons isoprene and α- and β-pinene Atmospheric Environment 16: 1017–1020. doi: 10.1016/0004-6981(82)90187-1
Resources
- Read about the beneficial effects of tree canopies: Guerrieri R (2019) The secret life of forests. Science in School 46: 20–24.
- Find out about the aircraft used for measuring atmosphere chemistry: Leather K et al. (2014) Up, up and away: using aircraft for atmospheric monitoring. Science in School 29: 9–12.
- Read more about how forests protect us from the effects of the climate crisis in this Guardian Article.
- Understand the role of the oceans in climate change: Harrison T, Khan A, Shallcross D (2017) Climate change: why the oceans matter. Science in School 39: 12–15.
- Read about the hole in the ozone layer and its evolution: Shallcross D, Harrison T (2010) A hole in the sky. Science in School 17: 46–53.
- Read which activities and technologies can help tackle climate change: Shallcross D, Harrison T (2011) Is climate change all gloom and doom? Introducing stabilisation wedges. Science in School 20: 60–65.
- Investigate the effect of fireworks on air quality with your class: Shallcross D, Harrison T (2011) Smoke is in the air: how fireworks affect air quality. Science in School 21: 47–51.
- Have your students make climate change predictions: Shallcross D, Harrison T (2008) Climate change modelling in the classroom. Science in School 9: 28–33.