Amber: an introduction to organic chemistry Teach article
Did you know that the electron and electricity are named after amber, the ‘gold’ of the Baltic Sea? Bernhard Sturm’s teaching unit based on this fossilised resin introduces not only conductivity but also many other characteristics of solid organic compounds.
Introduction
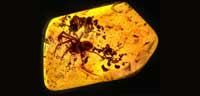
Image courtesy of Steev Selby;
image source: Wikimedia
Commons
Amber has been used as jewellery, as an ingredient in perfumes and in folk medicine for thousands of years – but it also has its place in science. It was the first substance on which electrostatic phenomena were observed, by the Greek philosopher Thales of Miletus, 600 BC, and it gave electricity its name: in 1601, the English physicist William Gilbert, the first to distinguish between magnetic and electrical attraction, coined the term ‘electricus’ for the property of attracting small objects after being rubbed, derived from amber’s Greek name elektron (meaning shiny).
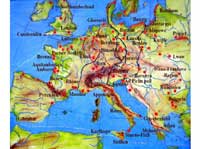
Amber discovery locations
in red, historical amber
routes in black and red,
rivers in blue.
Click to enlarge image
Image courtesy of Johannes
Richter; image source:
Wikimedia Commons
Amber is plant resin that fossilised either inside the plant, or after oozing out from it. Pieces of amber can be 20 to 320 million years old, but it is difficult to be certain: radiocarbon dating can only be used for specimens up to 50 000 years of age. So you have to determine the age of the surrounding sediment, which can be misleading because the amber may have originated far from the location in which it was discovered. Although amber is found all over the world, including in the Dominican Republic where rare blue amber is mined, possibly its most famous deposits in Europe are in the Baltic Sea, where large quantities are found. Yet amber is also found in eastern Europe, the North Sea, the Alps, northern Spain and Sicily. Pieces of amber torn from the sea floor are cast up by the waves and collected by hand, dredging or diving. Elsewhere, amber is mined, both in open works and in underground galleries.
The heterogeneous yellow to red organic macromolecule fossilises from two types of soft, sticky plant resin: terpenoid resins or phenolic resins. Terpenoid resins, produced by both conifers and angiosperms (flowering plants), consist of ring structures formed from isoprene (C5H8) units. Phenolic resins are found only in angiosperms, and include lignins, flavonoids and certain pigments.
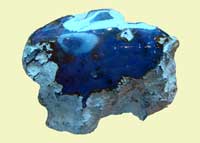
25-40 million years old
Public domain image; image
source: Wikimedia Commons
Resins protect injured plants from further damage, oozing out and hardening to form a defence against invading fungi and insects. The volatile fractions of resins are scented (think of the typical scent of pine resin), but it is the sticky, non-volatile, di- (C20) and tri-terpenoid (C30) fractions that fossilise into amber via free-radical polymerisation. During this maturation process, which takes place over millions of years, polymerisation, isomerisation, cross-linking and cyclisation may occur, forming a mixture of substances with the general formula C10H16O. A small amount of sulphur (up to 1%) may also be included.
Since amber has many classical properties of solid organic compounds, such as being combustible, not conducting electricity and being electrostatically chargeable, it is a good model substance to introduce these compounds in general, despite its varied and complex composition. It offers the added value of putting chemistry into a broader context, thus appealing to students who are not normally interested in the subject, because there are links to arts, biology, earth science and physics.
This five-lesson teaching unit is suitable for students aged 16+ who already know about density, conductivity and the electric circuit. You may want to provide textbooks from earlier grades for the students to refresh their knowledge. The teaching unit consists of six core activities: over four lessons of 45 minutes each, groups of students rotate through the stations, so that each group performs all the activities. Each activity will take about 20 minutes. In a large class, it may be useful to provide two of each station. In the final lesson, the students present their results in class.
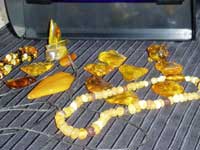
one are very useful for the
experiments
Image courtesy of Bernhard
Sturm
It is possible to extend the teaching unit with additional optional activities (see below), which may involve colleagues from other subjects. Alternatively, you may perform all or part of the activities as demonstration experiments or by the entire class simultaneously. The methods used in the different activities are quite varied, and the results tend to be easily remembered by the students.
The amber required for these activities can easily be obtained through online shops. One 30-35cm-long ‘baby chain’ for teething babies, available for about €8-20, will suffice to perform all core activities with about 30 students. Often, students are also keen to take family jewellery into class. The only experiment that will actually use up or damage the amber is combustion.
Core activities
For those students who are especially fast, you may want to provide further organic compounds on which they can perform the same experiments and compare to amber. These can be: for Experiment 2, other saturated organic compounds such as alkanes, e.g. in the form of a gas burner (with a yellow flame) and a lighter; for Experiments 3 to 6, plastics such as polyvinylchloride and polyethylene, as well as different types of wood (for example pine and oak, e.g. from a set of density cubes) and colophony (violin rosin, used to rub the hairs of the bow).
1) Geological origin
The students should compare the date and process of formation for natural deposits of amber, crude oil and coal by performing Internet and literature searches. They should critically evaluate the reliability of different sources of information and note down the dates and processes given in the different sources. For websites, they should note down the date at which they were accessed. See Table 1 for an example of what they may find.
Source of information | |||
---|---|---|---|
dtv-Lexikon, Munich, 1966 | http://en.wikipedia.org accessed on 31/03/2011 | ||
Crude oil | Time of origin | Cretaceous, 145-65 million years ago | Millions of years ago |
Formation | Small organisms sank to the bottom of the sea, forming sapropel (organic sludge); they were digested in the sediment under anaerobic conditions and high pressure. | Large quantities of prehistoric zooplankton and algae settled on the bottom of a water body under anoxic conditions; the organic matter mixed with mud and was buried under sediment; heat and pressure led to formation of crude oil. | |
Coal | Time of origin | Carboniferous, 360-300 million years ago | Carboniferous (359-299 million years ago) |
Formation | Dead tropical plants sank into the mud where they were covered by sand and clay; high pressure and anaerobic conditions led to carbonisation. | Layers of plant matter accumulated at the bottom of a body of water; mud or acidic water protected them from biodegradation and oxidisation; they were covered by sediment and metamorphosed into coal. | |
Amber | Time of origin | Devonian to Tertiary, 400-40 million years ago | Upper Carboniferous (320 million years ago) and later |
Formation | Resin oozed from trees to the ground, sank below the sea level after the climate changed, and polymerised under anaerobic conditions. | Resin that was either still in the plant or had oozed out and dropped to the ground, often acquiring impurities; high temperatures and pressures due to overlying sediments first led to formation of copal (an intermediate stage of polymerisation and hardening, between ‘gummier’ resins and amber); sustained heat and pressure drove off terpenes, resulting in amber formation. |
2) Combustion
Remind your students that high levels of carbon in a burning organic substance will lead to a sooty flame. The students should then hold a piece of amber (German Bernstein = Börnsteen = burning stone) with a pair of crucible tongs below a glass test tube, then burn the amber with a match and watch the soot collecting on the test tube.
To link the activity to the topic of particulate matter pollution by combustion engines, the students can vary the combustion conditions of a Bunsen burner by opening and closing the draft, and discuss how to avoid soot production.
Safety note
wear safety goggles and do not overheat the glass, as it may explode. Do not burn polyvinylchloride (available as optional material for Experiments 3 to 6), which would result in the production of harmful dioxins. See also the Science in School general safety note.
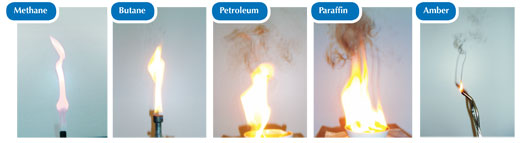
Images courtesy of Bernhard Sturm
3) Density
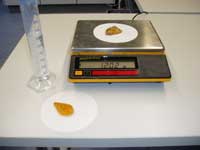
the balance) can be
distinguished by determining
their density.
Click to enlarge image
Image courtesy of Bernhard
Sturm
The students should determine the density of amber (1.050–1.096 g/ml), which is only slightly higher than that of water (about 0.998 g/ml at room temperature). For this experiment, more accurate results will be achieved using a larger piece of amber with no hole in it. I own a large piece of amber and a piece of flint of comparable size and like to give both to the students, asking them to determine which is which by measuring their densities.
You will need a piece of amber, a measuring cylinder, water (containing a small drop of washing-up liquid to reduce the surface tension and improve the accuracy of measurements) and a balance.
Weigh the piece of amber. Partly fill the cylinder with water and note down the volume of water. Add the amber and note the difference in volume. Calculate the density of amber as:
Density of amber [g/ml] = weight of amber [g] / (volume with amber [ml] – volume without amber [ml])
Many organic compounds have densities similar to water (0.8–1.2 g/ml). Polyvinylchloride is atypical, with a density of 1.4 g/ml, due to its heavier chloride atoms.
4) Separating amber from a mixture of organic and inorganic compounds
The students will learn how to separate amber from rocks and sand, an experiment with practical relevance for amber mining.
- Weigh an empty beaker.
- Add a defined volume of water and weigh it again to determine the mass of the water.
- Next add a mixture of sand, rocks and amber, and weigh the beaker again. Also note down the volume contained.
- Gradually add salt and mix until the amber floats. Weigh the full beaker again and note down the volume contained.
What is the density of the salt water? Calculate it as:
Density of salt water [g/l] = [(mass of beaker at the end (step 4) – mass of beaker before salt is added (step 3)) + (mass of beaker with water (step 2) – mass of empty beaker (step 1))] / [volume of water + (volume of beaker at the end (step 4) – volume of beaker without salt (step 3))]
This should of course be higher (>1.1 g/ml) than the density of amber determined in Experiment 3, otherwise the amber would not float.
The students should illustrate their ideas of how this technique could be developed into a technology for continuously mining amber (see image below).
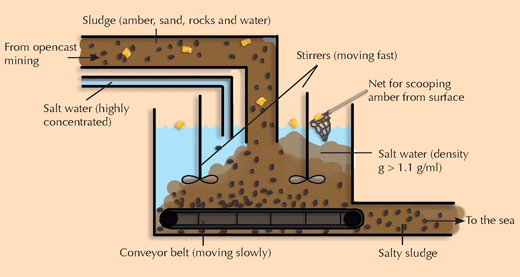
Image courtesy of Bernhard Sturm and Nicola Graf
5) Conductivity
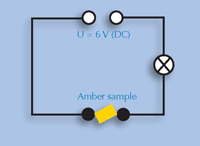
Sturm
The students will learn that solid organic compounds do not conduct electricity, by building an electric circuit using a power supply, three cables and a light bulb to test the conductivity of amber. If they are not familiar with the experimental setup, you could supply a physics textbook for them to look it up.
6) Charge separation
The students will learn about electrostatic induction and charge separation, performing Gilbert’s electrostatic experiment: they rub a piece of amber with wool and see that it attracts small pieces of paper or, for example, the dried pith from the centre of an elder (Sambucus spp.) twig. This also works well with the small pieces of amber from a baby chain.
The experiment will not work well if the air is humid, because water in the air will conduct electricity and reduce the electrostatic charge on the amber. Damp fingers will do the same; for better results, the students could use insulated (plastic) tweezers to hold the amber.
Optional activities
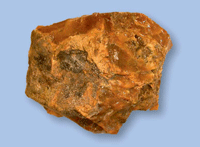
Image courtesy of V Girard / D
Néraudeau, UMR CNRS 6118
Making jewellery
To link art and chemistry and foster your students’ technical skills, you could get them to produce their own amber jewellery. You will need raw amber pieces of at least 15 mm in diameter. Sand the piece of amber with wet fine sandpaper (Coated Abrasive Manufacturers Institute grit size 120–1000), then polish it with toothpaste. Rinse with water and dry with kitchen roll, then rub in some cooking oil using a cloth. Pierce the amber with a hot needle (this should be done by the teacher) or drill a small 1-2 mm hole. Thread the amber onto a nylon or leather string to make a necklace or bracelet.
Links to biology
For a link to biology, the students can look at inclusions in amber and discuss tree resins in depth – what is their composition, where do they occur, what is their function and what is the structure of wood?
Classification of amber
Plant resins are so diverse that the distinct chemical composition is used to identify from which plant species a piece of amber formed. This does not mean that similar resins must originate from similar plants, however: recent research has revealed that resins of extremely similar molecular composition can be produced by entirely unrelated plants (Bray & Anderson, 2009) – the distinctions can be quite small. On the basis of their chemical constituents, five classes of amber are roughly defined:
- Class I: by far the most abundant, comprising carboxylic acids; three subclasses
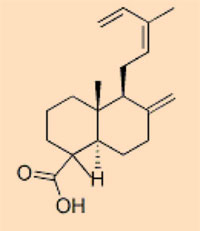
- Class II: formed from resins with a sesquiterpenoid base, such as cadinene
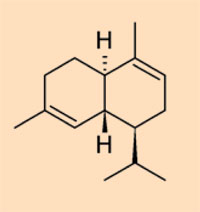
- Class III: natural polystyrenes
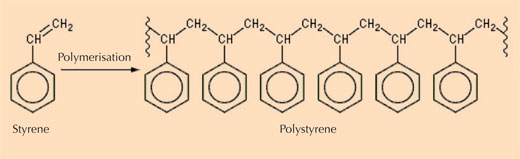
- Class IV: a collection of non-polymerised ambers which consist mainly of cedrane-based sesquiterpenoids
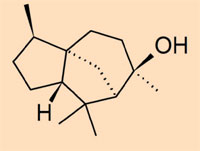
- Class V: considered to be produced by a pine or pine relative; a mixture of diterpenoid resins and n-alkyl compounds (R-NH-CH3)
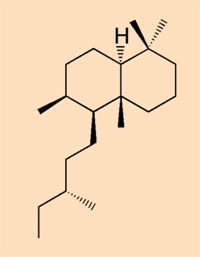
Public domain images; image source: Wikimedia Commons; adapted by Nicola Graf
Amber research at ESRF
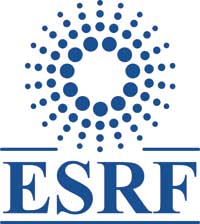
Pieces of amber are a rich source of fossil evidence. At the European Synchrotron Radiation Faciliy (ESRF)w1 in Grenoble, France, powerful X-rays are used to study inclusions in amber. This is especially useful for opaque amber pieces, which are inaccessible to palaeontologists with classical microscopy techniques. Several hundred animal inclusions from the mid-Cretaceous, 100 million years ago, have been identified.
In another study at ESRF, researchers used the same technique to obtain detailed three-dimensional images of feathers enclosed in translucent amber, which may have belonged to a feathered dinosaur – an intermediate in the evolution to modern birds.
For more information, read the online reportw2 from ESRF, one of the members of EIROforum, the publisher of Science in School.
References
- Bray PS, Anderson KB (2009) Identification of carboniferous (320 million years old) class Ic amber. Science 326(5949): 132-134. doi: 10.1126/science.1177539
- The free full-text article is available at: www.sciencemag.org/content/326/5949/132.short
- For drama activities in the chemistry and physics classroom, including one about the radical polymerisation of ethene to polyethylene in class, see:
- Sturm B (2009) The drama of science. Science in School 13: 29-33.
Web References
- w1 – For more information on ESRF, see: www.esrf.eu
- w2 – Scientists at ESRF used powerful X-rays to study amber inclusions. See: www.esrf.eu/news/general/amber
Resources
- To download charts of Earth’s history, see: www.stratigraphy.org/upload/ISChart2009.pdf or www.chronos.org/downloads/timetowerparis_highres.png
- To learn about research into biodegradable plastics, see:
- Bradley D (2007) Plastics, naturally. Science in School 5: 66-69.
Institutions
Review
Bernhard Sturm, who has already published another enjoyable article in Science in School (Sturm, 2009), is a model of creativity in the field of science teaching.
Those who think that chemistry and physics are boring subjects should try the activities based on amber proposed by the author. Starting from this ancient material and following the suggested links, a science teacher can explore many different topics related to amber and discover unexpected relationships with arts and humanities.
The different core activities, in fact, provide the opportunity to address organic chemistry (natural and man-made polymers), earth science (sedimentary rocks, fossils, fossil fuels), physics (density, separation methods, conductivity and charge separation), environmental science (combustion, pollution) and biology (plant resins, amber inclusions).
For those interested in interdisciplinary links, the choice is also wide: arts and crafts (making jewellery), history (the Amber Road, the Amber Room) or economy (amber mining and commerce), just to mention some.
Finally, a teacher only needs to get some pieces of the ‘gold of the Baltic Sea’ (which is –luckily – much cheaper than true gold) to follow Bernhard Sturm in the footsteps of Thales of Miletus, William Gilbert and others.
The article might provide valuable background reading for a visit to a natural history or science museum and can also be used as a comprehension exercise. Possible questions include:
- To assess the age of a piece of amber:
- radiocarbon can normally be used
- it is necessary to date the surrounding sediments
- different methods are used depending on the circumstances.
- Which of the following materials is most different in density compared to amber?
- polyethylene
- polyvinylchloride
- oak wood
- colophony
Giulia Realdon, Italy