Alec Jeffreys interview: a pioneer on the frontier of human diversity Understand article
Professor Sir Alec Jeffreys, the inventor of DNA fingerprinting, remembers his childhood passion for science, explains what we have learned from direct DNA analysis, and describes his work with Chernobyl survivors. Interviewed by Russ Hodge and Anna-Lynn Wegener from the European Molecular Biology…
What attracted you to science in the first place?
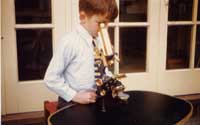
his new microscope
I was born a curious child; I was the sort of child who would go around slicing up earthworms to see what was inside – a fairly ghastly child. But at the age of eight, I got two great things from my dad: a microscope and a chemistry set. And not any ordinary set; this was a real, fully lethal chemistry set.
Basically, I was a self-taught organic chemist. By the age of 11 or 12, through sheer enthusiasm, I’d taken myself up to first-year university level. There were bangs, there were stinks, I was making the most extraordinary compounds and you learn very rapidly if you’re doing that sort of practical science. It’s a way of getting immersed in science which today is absolutely impossible because of health and safety concerns.
And nothing happened at school to distract you or put you off science?
I had the most fantastic teachers, particularly my biology teacher, Mr Barton. I’d say, “I want to know what a starfish looks like inside,” and he’d get me a dead starfish so I could dissect it. So the school was enormously important, but they were working on a child who had already been converted – at that point, I was completely addicted to science anyway.
You’ve been studying genetics and human variation for nearly three decades now. What do we know now about human variation that we didn’t know thirty years ago?
We first had the tools for beginning to look at human variation in late 1977. Before that, what we knew was based on indirect inference. We could look at proteins in the blood or in saliva, and by studying variations in the products of genes, we could make inferences about variation in the genes themselves. Thirty years ago, though, we had no inkling of the true level of variation at that most fundamental level of all: the DNA level. Within about two years, though, it became clear that there were going to be many, many millions of sites of differences between two people.
Hand in hand with the Human Genome Projectw1, there’s been the parallel Human Genome Diversity Projectw2. We’ve learned not only the true extent of variation, but also a great deal about how that variation is distributed between different populations, and that most genetic variation is shared by everybody on the planet. That simply reflects the fact that we’re a young species which has not had enough time to split into different, genetically distinct, subspecies. The project has also told us that Africa is the richest source of genetic diversity, which is consistent with mankind having evolved in Africa. Again, these are insights that simply couldn’t have been achieved without looking at the DNA.
But the other thing that direct analysis of DNA has taught us is that the old model for how DNA varies from one person to another was radically wrong. DNA was seen as just a string of letters, with individuals differing in terms of single letter changes, the so-called single nucleotide polymorphisms, or SNPs. We now know not only that there are many sites of these single nucleotide polymorphisms, but also that the human genome is full of little deletions, duplications, inversions, all sorts of rearrangements. There are even little segments of DNA that can copy themselves to a new location – the so-called transposable elements. These little molecular fleas, hopping around on the human genome host, make up about 25-30% of the entire human genome.
There are also regions of repeat segments of DNA, known as minisatellites. These are my favourites and they’re the ones that underpin DNA fingerprintingw3. They really interest me because some of them are fantastically variable, hence their use in DNA fingerprinting. Not only that, but some of them can be major causes of inherited disease, such as Huntington’s Disease, which is caused by repeat segments of DNA replicating and making toxic gene products, killing cells in the brain. We can also look at the origin of inherited disease itself: we can take the most common cystic fibrosis mutation and begin to estimate when it arose in human history and how it spread.
So direct analysis of DNA has told us about normal variation, pathological variation and the origins of pathological changes; it’s created entirely new types of genetic marker, such as those used in forensic DNA fingerprinting, and it’s told us quite a lot about the overall evolution of the species.
This whole field is now moving really fast. What do you expect will be the kinds of new issues that we’ll be able to deal with in the next decade?
Many of the issues that we’re going to be talking about ten years from now haven’t even been thought about now. Having said that, it’s obvious that some things are going to be important, such as the genetic basis of common diseases like diabetes. Diabetes has a genetic component but it’s very difficult to know which of all the millions of sites of genetic variation directly affect your risk of developing the disease. The environmental pathways are absolutely obvious – lack of exercise and lousy diet – and that can be changed right now: people can dramatically modify their risk of diabetes without even thinking about genetics. But genetics would give insights into the mechanisms, and could lead to new drugs to minimise an obese person’s risk of developing diabetes.
Could you explain the distinction between DNA fingerprinting and the types of profiling that are used to try to anticipate future disease?
With old-fashioned DNA fingerprinting, you couldn’t distinguish between ethnic groups, or even predict things like hair colour and eye colour, or disease liability. With modern forensic DNA typing systems, that’s not quite true: there are some weak hints about ethnicity and some very, very weak hints about specific disease liabilities, particularly about diabetes.
People have said, “Why don’t you create very high-throughput DNA-typing systems to help the police in their enquiries and simultaneously identify people at risk of disease and help them make the right lifestyle decisions?” My view is that the police use of DNA and the medical use of DNA should be totally, completely and utterly separate. I would be content for the police to have a sample of my DNA if it were to help in a specific investigation. I would not be happy for the police to look at characters in my DNA that are important to me as an individual – that’s an invasion of my genetic privacy. As soon as you cross the boundary between the forensic and medical uses of DNA, I think you are creating a seriously difficult situation.
That would undermine the great success story of the forensic use of DNA. The public by and large are content for the police to retain samples of DNA from convicted people, analyse them and create databases. That sympathy would start evaporating if the police had all sorts of information on disease liabilities, ethnic origins of people, and family relationships.
You’ve been involved with some work involving Chernobyl. Could you explain what you’ve been doing and what’s come out of that work?
This came straight out of DNA fingerprinting. The repeat segments of DNA we use for DNA fingerprinting are highly variable because they are unstable, so as they are transmitted from parent to child, they quite often spontaneously change – which is very different from an average human gene. If you were to use a typical gene, you might have to look at 10 000 children before you found a new mutation. With these bits of DNA, in the most extreme example, about one child in four carries a new version. So they’re very easy to pick up.
Given that instability, a colleague of mine, Yuri Dubrova from Moscow, came up with the idea of using these DNA segments for mutation monitoring. For example, in Chernobyl, where there was a mass release of radioactivity into the environment, can you detect radiation-induced mutations in children? We did some of the experiments in an animal model system, and showed that if you irradiate mice, more mutations accumulate in the repeat segments of DNA in their offspring. So these bits of DNA are not only spontaneously highly unstable, they are also exceedingly sensitive to radiation, and give us a very simple way to pick up radiation-induced mutations.
Having shown that, Yuri then went on to parallel studies in Chernobyl, which blew up in April 1986. He recruited mother-father-child trios in Belarus, where the child had been born some time after the disaster, where both parents had been permanently living in the area, and where there was information available on the level of radiation in the environment. Again, he found that the children had an elevated number of these mutations and that the elevation seemed to be roughly in proportion to the amount of radioactivity in the environment.
Now if that is true, that is the first direct evidence in humans for radiation-induced heritable mutations. Big genetic studies of the survivors of Hiroshima and Nagasaki lacked the power to be able to ask those sorts of questions. We also saw these mutations in both mice and humans at levels of radiation exposure that were traditionally thought to be without significant genetic risk. That may well question the basis for setting radiation thresholds and for risk estimation.
In mice, we also saw a trans-generational phenomenon, whereby if you expose a mouse to radiation, you see new radiation-induced mutations appearing not only in its offspring, but also in its offspring’s offspring and in their offspring, even though they’ve never been exposed to radiation. We don’t know if that happens in humans, but if it does, then it raises issues about the effects of radiation – not just for individuals, but for their unexposed children, grandchildren, and more distant descendents. If that phenomenon works in a trans-generational way, as it does in mice.
Is there still debate about what the results of this study mean?
Yes, I have to stress that there is no consensus yet on the human response. I mean, there is a response, but this is epidemiology and one thing you cannot do with population studies is define cause and effect. So you can always argue that maybe it’s got nothing to do with radiation, that maybe the people living in the most seriously contaminated areas were so nervous that they smoked a lot and that it was the smoking that induced the mutations. You can see correlations, but to really pin down cause and effect, to really see if radiation is the cause, is very difficult.
The most direct experiment that we subsequently did – which shows just how confusing this particular field can be – was to collect semen from men and count the number of sperm that had the mutations. We then irradiated these men’s testes, waited a while and then collected and analysed further semen samples. After radiation, we found no effect on mutation rate whatsoever!
You might wonder how we got anyone to volunteer. We worked with testicular cancer patients, so we needed to find a patient who’s not only going to undergo radiotherapy – hence the testis irradiation – but is also prepared to give semen samples. Worldwide we found three people who were prepared to do that. However, despite the small size of the study, there was clearly no effect.
So to summarise, we found radiation-induced heritable mutations in Belarus, and when the study was repeated in the northern Ukraine, we saw the same effect. At a nuclear weapons test site in Kazakhstan, with contaminated villages, the same effect was seen. Studies on survivors of the Japanese atom bombs showed no effect. Direct exposure of the testes also showed no effect. And studies of the Chernobyl clean-up workers and their families likewise showed no effect. So there’s no consensus yet – and that’s the way science works.
Web References
- w1 – Wikipedia entry on the Human Genome Project
- w2 – Wikipedia entry on the Human Genome Diversity Project
- w3 – Wikipedia entry on DNA fingerprinting
Resources
- Alec Jeffreys’ website
- Wikipedia entry on Alec Jeffreys
- The International HapMap Project is an effort to develop a haplotype map of the human genome, to identify and catalogue genetic similarities and differences in human beings.
Review
As a teacher, I was particularly impressed by the first part of the article, in which Professor Jeffreys remembers his fantastic science teacher. This is a real reward for good science teachers.
Immacolata Ercolino, Italy