EIROforum: introducing the publisher of Science in School Understand article
EIROforum Click to enlarge image EIROforumw1 is a collaboration between seven European inter-governmental scientific research organisations. The organisations focus on very different types of research – from molecular biology to astronomy, from fusion energy to space science. They use very…
EIROforum
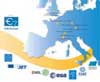
EIROforumw1 is a collaboration between seven European inter-governmental scientific research organisations. The organisations focus on very different types of research – from molecular biology to astronomy, from fusion energy to space science. They use very different techniques – including enormous particle accelerators, beams of neutrons or high-energy X-rays, large telescopes or the International Space Station. Nonetheless, the seven organisations share a common structure and – as EIROforum – a common mission.
Each of the organisations (known as EIROs) is funded by member states, which share the costs and can send their scientists to use the facilities. By combining the financial resources of many countries, these organisations provide equipment and facilities far more sophisticated than any one country could afford, enabling world-class scientific and technological research in interdisciplinary fields.
As EIROforum, the EIROs come together to support European science by sharing their experience, resources and facilities. Together, they interact with the European Commission and other organs of the European Union, national governments, industry, science teachers, students and journalists.
By co-operating on large-scale outreach activities, EIROforum communicates the importance and fascination of science to a wide audience. Through Science in School, for example, EIROforum motivates and encourages young people to explore scientific subjects, and shows them that science is a rewarding career.
CERN, the European Organization for Nuclear Research
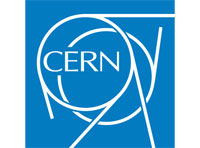
CERNw2 is the world’s largest particle physics laboratory, and is based in Geneva, Switzerland. Research focuses on fundamental physics: using some of the world’s largest and most complex scientific instruments to study the basic constituents of matter – the fundamental particles. By studying what happens when these particles collide, physicists learn about what the Universe is made of and how it works.
The instruments used at CERN are particle accelerators and detectors. Accelerators boost beams of particles to high energies before they are made to collide with each other or with stationary targets. Detectors observe and record the results of these collisions. The newest of CERN’s instruments is the Large Hadron Collider (LHC), a 27 km long particle accelerator spanning the French-Swiss border.
Does particle physics have its head in the clouds?
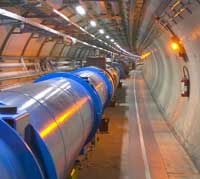
Image courtesy of CERN
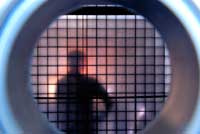
Image courtesy of CERN
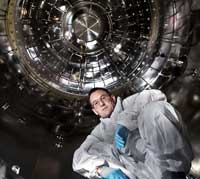
Image courtesy of CERN
While the LHC is delivering its first beams for experiments, the 50-year-old Proton Synchrotron is being used to investigate Earth’s climate.
Although many experiments worldwide are investigating what affects the planet’s climate, the CLOUD experiment at CERN is the only one that makes use of a particle accelerator. In the experiment, a beam of protons is used to simulate the difference of particle flux in the atmosphere, which varies by a factor of 100 from ground level to the outermost layers of the stratosphere.
From the Proton Synchrotron, protons enter the experiment’s 3 m diameter aerosol/cloud chamber, which accurately recreates various conditions in the atmosphere. The chamber is fitted with an array of sensitive analytical instruments to follow the processes involved: the birth of embryonic aerosols, and their growth to become the seeds for cloud droplets and ice particles. CLOUD will also study the effect of cosmic rays on the cloud droplets and ice particles.
CLOUD is unique in that the parameters that are likely to affect cloud formation can be controlled in the laboratory. CLOUD scientists can vary the chemicals involved and the temperatures of the gas in the chamber. The temperature in the cloud chamber can be varied from -90 °C to +40 °C, which essentially covers the range of atmospheric temperature from the coldest part of the stratosphere to the warmest part of the troposphere.
Ultimately, by modelling how cloud processes affect climate, the CERN scientists and their collaborators hope to be able to investigate possible additional causes of climate change.
For more information, see Erlykin et al. (2010), CERN (2009), the CLOUD websitew3, and an online lecture about the CLOUD experimentw4.
The European Fusion Development Agreement – Joint European Torus (EFDA-JET)
EFDA-JETw5 is Europe’s fusion experiment, based at Culham Science Centre in the UK. JET is the world’s largest tokamak – a device that uses hugely powerful magnetic fields to confine a very hot gas or plasma so that the hydrogen nuclei in the plasma fuse together to release energy.
The world’s largest fusion experiment, JET is currently the only machine that has created fusion – with temperatures reaching an amazing 150 000 000 °C in the centre of its plasma. At the forefront of research to exploit fusion for commercial electricity generation, JET focuses on key issues for its successor, ITERw6, an international tokamak experiment being built in France.
Another brick in the wall
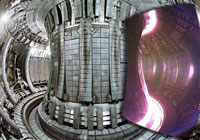
superimposed image of an actual
JET plasma
Image courtesy of EFDA-JET
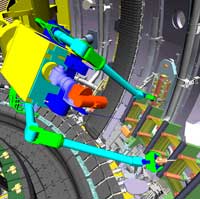
simulation used by JET robotics
group
Image courtesy of EFDA-JET
In fusion devices such as JET, components close to the plasma are constantly bombarded by heavy bursts of heat and neutrons from the turbulent, writhing plasma as it tries to escape from its magnetic cage. Some components have to withstand peak temperatures of more than 1000 °C, despite being actively cooled. As a result, potential damage to plasma-facing walls is a real challenge for fusion research and technology, and identifying new durable materials is crucial in progress towards fusion power plants of the future.
As part of a €60 million enhancement programme, JET is being fitted out with a new internal wall made of the same materials that ITER will eventually use. This is a mammoth undertaking. During all of 2010, more than 4500 carbon fibre composite components that line the inner wall of the machine will be replaced by beryllium- and tungsten-coated components, testing the robotic capability of JET’s remote handling systems to its limits.
But when operations restart in 2011, the revamped machine will have the capability of going far beyond current performance levels. The upgraded JET has an ambitious aim: to increase the world-record 16 MW of fusion power produced back in 1997 to 30 MW.
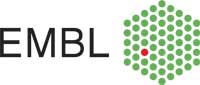
The European Molecular Biology Laboratory (EMBL)
EMBLw7, with its main laboratory in Heidelberg, Germany, is dedicated to basic research covering the full spectrum of molecular biology at all levels, from the molecule to the organism, as well as computational biology, bioinformatics and systems biology.
Many scientific breakthroughs have been made at EMBL, most notably Christiane Nüsslein-Volhard and Erich Wieschaus’s discovery of important genetic mechanisms that control early embryonic development, for which they were awarded the Nobel Prize in Physiology or Medicine in 1995w8.
Gender-bending mice: insights into sex determination
In humans and most other mammals, an individual’s sex is determined by its sex chromosomes: females have two X chromosomes, and males have an X and a Y. Scientists had long assumed that the female pathway – the development of ovaries and all other female traits – was the default: if an embryo had a gene called Sry, which is located on the Y chromosome, it would develop into a male; if not, then the result would be a female.
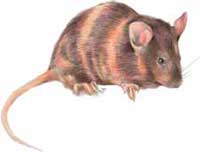
Nadia Rosenthal
Image courtesy of Nadia Rosenthal
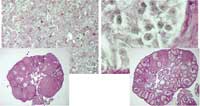
mouse, with close-up showing the
typical female granulosa cells (right),
which, when Foxl2 was silenced (left),
took on characteristics normally found
in testes. Click to enlarge image
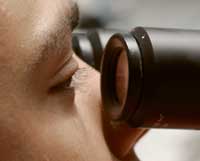
Image courtesy of EMBL
A gene called Foxl2, which is located on a non-sex chromosome and therefore present in both sexes, was known to play an important role in the female pathway, but its precise function remained elusive. When EMBL scientists and their UK collaborators turned off this gene in the ovaries of adult female mice, they found that cells in the ovaries turned into cells typically found in testes.
“We were surprised,” says Mathias Treier, one of the EMBL scientists. “We expected the mice to stop producing oocytes, but what happened was much more dramatic: …cells which support the developing egg took on the characteristics of cells which usually support developing sperm, and female hormone-producing cells switched to the male type.” This challenges the long-held assumption that the development of female traits is a default pathway, showing that the male pathway needs to be actively suppressed, and also grants a valuable insight into how sex determination evolved.
These findings will have wide-ranging implications for reproductive medicine and may help to treat sex differentiation disorders in children or to understand the masculinising effects of menopause on some women.
For more information, see the original publication in Cell (Uhlenhaut et al., 2009) and Mathias Treier’s online presentationw9.
The European Space Agency (ESA)
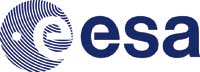
ESAw10 is Europe’s gateway to space. From its headquarters in Paris, France, ESA runs programmes to find out more about Earth, its immediate space environment, our Solar System and the Universe, as well as to develop satellite-based technologies and services, and to promote European industries.
Together with the US National Aeronautical and Space Administration (NASA), the Russian Federal Space Agency (Roscosmos), the Canadian Space Agency (CSA) and the Japan Aerospace Exploration Agency (JAXA), ESA operates the International Space Stationw11.
‘Sugar-cube’ sensors to monitor Earth’s orientation
One of ESA’s future Earth observation missions will monitor Earth’s orientation in space with the help of the smallest gyro ever flown by Europe. Now being tested, the sugar cube-sized device at the heart of the gyro unit is derived from a sensor used in anti-lock braking systems on millions of cars: micro-electro mechanical systems (MEMS).
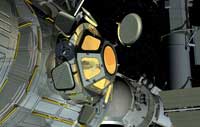
observation module of the
International Space Station
Image courtesy of ESA / D Ducros
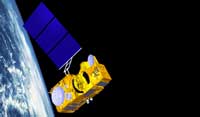
Sentinel-3 satellite, scheduled to
be launched in 2013, which will
provide global ocean, ice and land
vegetation observations
Image courtesy of ESA
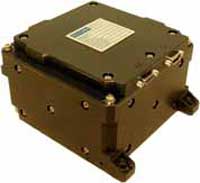
Image courtesy of ESA
There is no up or down in space. Satellites track their pointing direction using the same approach that is used onboard submarines and aircraft: fast-spinning gyroscopes that maintain a fixed orientation in the same way as a child’s spinning top. But space-quality gyros employing this principle are complex, bulky and insufficiently reliable for long space missions.
For ESA, therefore, MEMS are an especially promising innovation. They are made in a similar way to microprocessors but incorporate moving parts or sensors, enabling complete devices to be fitted onto a single silicon chip.
Attractive for space because of their small size, low power consumption and resistance to vibration, these micro-machined devices may sound exotic, but MEMS sensors are already used in their millions on European roads. In the last 15 years, the automobile industry has adopted MEMS in a major way. The devices are embedded throughout modern cars: MEMS accelerometers trigger airbags, MEMS pressure sensors check tyres and MEMS gyros help to prevent brakes locking and maintain traction during skids. And soon there will be MEMS in space, helping to monitor the orientation of Earth. Not bad for something the size of a sugar cube.
To find out more about the use of MEMS in Earth observation, visit the ESA websitew12.
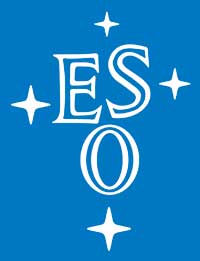
The European Southern Observatory (ESO)
ESOw13 is the foremost inter-governmental astronomy organisation in Europe and the world’s most productive astronomical observatory. From its headquarters in Garching, Germany, ESO operates three world-class observing sites in Chile: La Silla, Paranal and Chajnantor. At Paranal, ESO operates the Very Large Telescope, the world’s most advanced visible-light astronomical observatory, and VISTA, the world’s largest survey telescope. ESO is the European partner of a revolutionary astronomical telescope, ALMA, the largest astronomical project in existence, which is being constructed in Chajnantor. ESO is currently planning the European Extremely Large optical/near-infrared Telescope, the E-ELT, which will become ‘the world’s biggest eye on the sky’.
Orion in a new light
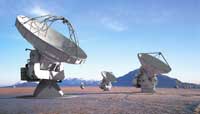
ALMA (ESO / NAOJ / NRAO) /
L Calçada / H Heyer / H Zodet
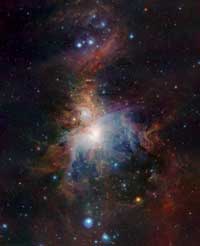
Nebula. Click to enlarge image
The Orion Nebula reveals many of its hidden secrets in a dramatic image taken by ESO’s new VISTA survey telescope. The telescope’s huge field of view can show the full splendour of the whole nebula and VISTA’s infrared vision also allows it to peer deeply into dusty regions that are normally hidden and to expose the curious behaviour of the very active young stars buried there.
VISTA – the Visible and Infrared Survey Telescope for Astronomy – is the latest addition to ESO’s Paranal Observatory. It is the largest survey telescope in the world, and is dedicated to mapping the sky at infrared wavelengths. The large (4.1 m) mirror, wide field of view and very sensitive detectors make VISTA a unique instrument. This dramatic new image of the Orion Nebula illustrates VISTA’s remarkable powers.
The Orion Nebula is a vast stellar nursery lying about 1350 light-years from Earth. Although the nebula is spectacular when seen through an ordinary telescope, what can be seen using visible light is only a small part of a cloud of gas in which stars are forming. Most of the action is deeply embedded in dust clouds, and to see what is really happening, astronomers need to use telescopes with detectors sensitive to the longer-wavelength radiation that can penetrate the dust. VISTA has imaged the Orion Nebula at wavelengths about twice as long as can be detected by the human eye.
For more information, see the VISTA web pagesw14.
The European Synchrotron Radiation Facility (ESRF)
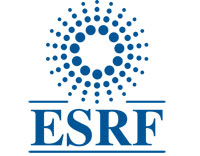
ESRFw15 operates the largest facility for research with X-rays in Europe. Each year, 7000 researchers travel to Grenoble, France, to conduct some 1000 different experiments, all at the cutting edge of modern science. Physicists work side-by-side with chemists and materials scientists. Biologists, medical doctors, meteorologists, geophysicists and archaeologists have become regular users. Industrial applications are also growing, notably in the fields of pharmaceuticals, cosmetics, petrochemicals and microelectronics.
More sustainable plastic bags – using X-rays
In early July 2010, the ESOF meeting in Turin, Italy, will coincide with an unusual experiment at ESRF: Dutch and Italian scientists will arrive in Grenoble with a machine for manufacturing thin films such as plastic bags. The objective is to observe, in situ, how the molecular structure of thin plastic film forms, with a view to using smaller quantities of polyolefins, materials synthesised from natural gas and oil, in plastic products of the future.
Plastic films are manufactured by extrusion: plastic granulate is melted and then forced through a die (a specially designed spout that determines the shape of the extruded polymer). The die used for carrier bags differs from a standard extrusion die in that it is cylindrical and has an air ring at the top which distributes an even flow of cool air inside the cylinder. As the plastic is extruded, it forms a tube into which cold air enters through the air ring, causing the tube to expand into a bubble. The air will also cool the plastic as it is moved upwards and rolled up as a double sheet, still sealed at both sides. To produce bags, the plastic films are sealed at one end and perforated at the other end across their width (think of a roll of rubbish bags).
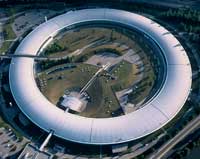
Image courtesy of Denis Morel / ESRF
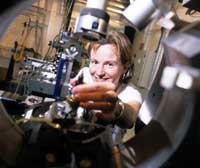
Image courtesy of G Duvernay /
Insign Studio / ESRF
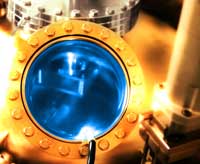
Image courtesy of S Evans / G Garner
The quality of the bag depends on how the molten plastic solidifies as it expands and cools. At ESRF, the process of solidification will be observed with X-rays while the plastic film is being processed. The scientists will use two experimental methods: wide-angle diffraction delivers information on the structure of the crystalline fraction of the plastic polymer, whereas small-angle scattering is sensitive to the long-range order due to alternating amorphous and crystalline regions. These methods can be used in real time, while the polymer is passing from the liquid to solid state. Both the degree of crystallinity as well as the extent of the long-range order are related to macroscopic properties such as elasticity and strength, which allows the manufacturing process to be optimised, resulting in stronger but thinner plastic films and ultimately saving raw materials.
It is estimated that enough plastic film is produced and used each year to wrap our planet in three layers of polymer film. Polyolefin films will remain part of our daily life for the foreseeable future, so even small savings in their manufacture would lead to a significant reduction in natural gas and oil consumption.
To see for yourself how plastic bags are manufactured, watch a video from Stanford University onlinew16.
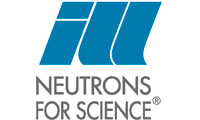
The Institut Laue-Langevin (ILL)
Based in Grenoble, France, ILLw17 operates one of the most intense neutron sources in the world. As a service institute, ILL makes its facilities and expertise available to visiting scientists: every year, some 1200 researchers and 800 experiments from more than 40 countries. Research focuses primarily on fundamental science in a variety of fields, including condensed matter physics, chemistry, biology, nuclear physics and materials science.
Whereas some visiting scientists are working on engine designs, fuels, plastics and household products, others are looking at biological processes at the cellular and molecular level. Still others may be elucidating the physics that could contribute to the electronic devices of the future. ILL can tailor its neutron beams to probe the fundamental processes that help to explain how our Universe came into being, why it looks the way it does today, and how it can sustain life.
Cutting-edge science: neutrons to the aid of European industry
Stone cutting is nothing new – even our prehistoric ancestors knew how to cut stones. Nonetheless, this ancient industry has recently come under scrutiny from scientists using very modern techniques: beams of high-energy neutrons.
Like many other European industries, Europe’s stone-cutting industry is under threat from cheaper international competition. To help protect more than 500 000 jobs, therefore, the EU has launched a research project (Pro-Stone) to develop better, longer-lasting stone-cutting tools.
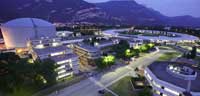
(the white ring at the back)
Image courtesy of ILL / Peter Ginter
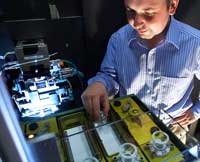
Image courtesy of ILL /
Alexis Chezière
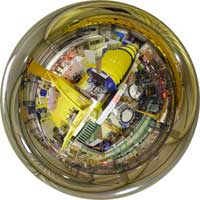
scattering instrument D11
Image courtesy of ILL / Artechnique
Stone is cut with fast-rotating steel discs. During use, these discs get very hot and are subject to high and uneven stress, which can cause them to suffer metal fatigue and to crack. As part of the Pro-Stone project, the effectiveness of incorporating titanium-nickel (TiNi) alloy inserts into the discs was tested. When the disc reaches a particular temperature, the ‘smart’ alloy insert is activated, exerting a large, compressive stress close the cutting edge of the disc, which should prevent any cracks from growing and protect the disc from metal fatigue.
Researchers at ILL and their Czech collaborators used high-energy beams of neutrons to map the three-dimensional stresses around the inserts. The stress was investigated both at ambient temperature and at 130 °C, above the activation temperature of the alloy insert. The results show that the insert causes a dramatic change in the stress experienced by the stone-cutting disc.
Through careful placement of smart inserts in cutting discs, therefore, it will be possible to improve the performance and lifetime of stone-cutting tools, giving the EU stone-cutting industry a new, competitive edge.
For further details of this research, see the Pro-Stone websitew18.
To learn more about stress, how it can be applied and how it is studied at ILL, see Hughes (2007).
References
- CERN (2009) On CLOUD nine. CERN Bulletin 24: 1-2. http://cdsweb.cern.ch/record/1180849
- Erlykin A et al. (2010) Cosmic rays, climate and the origin of life. CERN Courier 50(2): 15-17. http://cerncourier.com/cws/article/cern/41723
- Uhlenhaut NH et al. (2009) Somatic sex reprogramming of adult ovaries to testes by Foxl2 ablation. Cell 139(6): 1130-1142. doi: 10.1016/j.cell.2009.11.021
Web References
- w1 – For more information about EIROforum, see: www.eiroforum.org
- w2 – To learn more about CERN, see: www.cern.ch
- w3 – Visit the website of the CLOUD experiment at CERN: http://cloud.web.cern.ch/cloud
- w4 – For a video of Jasper Kirkby’s recent lecture ‘Cosmic rays and climate’, see: http://indico.cern.ch/conferenceDisplay.py?confid=52576
- w5 – More information about EFDA-JET is available here: www.jet.efda.org
- w6 – To learn more about ITER, the next step towards commercially available fusion energy, see: www.iter.org
- w7 – For more details about EMBL, see: www.embl.org
- w8 – To learn more about the work for which Christiane Nüsslein-Volhard and Erich Wieschaus were awarded the Nobel Prize in Physiology or Medicine in 1995, see: http://nobelprize.org/nobel_prizes/medicine/laureates/1995
- w9 – For an online video of Mathias Treier explaining how ovaries were turned into testes in mice, see: www.youtube.com/watch?v=-oL7RKUNchY
- w10 – To learn more about ESA, visit: www.esa.int
- w11 – Find out more about the International Space Station here: www.esa.int/esaHS/ESA0I6KE43D_iss_0.html
- w12 – For more information about ESA’s ‘sugar-cube’ gyro sensors, see: www.esa.int/esaCP/SEMVXYUHYXF_index_0.html
- w13 – For more information about ESO, see: www.eso.org
- w14 – To learn more about VISTA, ESO’s new telescope, see: www.eso.org/public/teles-instr/surveytelescopes/vista
- w15 – To learn more about ESRF, visit: www.esrf.eu
- w16 – Stanford University has produced a video showing the manufacture of plastic bags: www.youtube.com/watch?v=fte32FKRG9I
- w17 – For more information about ILL, see: www.ill.eu
- w18 – To learn more about the Pro-Stone project, see: www.dappolonia-research.com/prostonecm/doceboCms
Resources
- To find out how to build and use your own cloud chamber, see:
- Barradas-Solas F, Alameda-Meléndez P (2010) Bringing particle physics to life: build your own cloud chamber. Science in School 14: 36-41.
- To learn more about the LHC, see:
- Landua R (2008) The LHC: a look inside. Science in School 10: 34-45.
- Landua R, Rau M (2008) The LHC: a step closer to the Big Bang. Science in School 10: 26-33.
- To learn more about the International Space Station, see:
- Hartevelt-Velani S, Walker C (2008) The International Space Station: a foothold in space. Science in School 9: 62-65.
- Hartevelt-Velani S, Walker C, Elmann-Larsen B (2008) The International Space Station: life in space. Science in School 10: 76-81.
Institutions
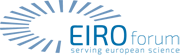