Recovering Pompeii Understand article
Do your students find it hard to see the application of science to other subjects? Montserrat Capellas from the European Synchrotron Radiation Facility in Grenoble, France, explains how modern chemical analyses are shedding light on ancient Pompeii.
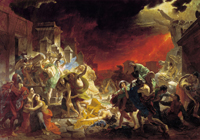
by Karl Pavlovich Briullov
Public domain image; Image source:
Wikimedia Commons
Artists in ancient Pompeii, Italy, painted the town red 2000 years ago with a brilliant crimson pigment that dominated many of the doomed town’s wall paintings. On 24 August 79 AD, the volcano Vesuvius erupted, burying nearby towns in pumice and ash. Instead of destroying Pompeii, however, it preserved it and the bodies of its inhabitants under the earth. Included in the World Heritage List by UNESCO in 1997, the ancient town of Pompeii is now the most visited archaeological site in Europe.
The Villa Sora, in the nearby town of Torre del Greco, remained buried until 20 years ago, when excavation works revealed the building. Since the discovery of the remains of the house, the distinctive red colour of the wall frescoes has turned black in many places, in a rapid degradation process that is not yet well understood scientifically. The source of the red colour in Torre del Greco and Pompeii is mercury sulphide (HgS), commonly named cinnabar. This is a deep red pigment used extensively to cover the background of paintings, giving a uniform red background to pictures. Yet, in certain circumstances, it can become unstable, turning into dull grey-black shades.
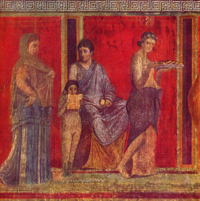
Scientists have been wondering for many years why the red walls in Pompeii were turning black. As early as the 1st century BC, Vitruvius, in his treatise De Architectura, mentioned the problem, and gave the recipe for a sort of protective varnish based on Punic wax (a waxy soap made of beeswax and soda lime): “Those who are desirous that the vermilion should retain its colour, should, when the wall is coloured and dry, rub it with a hard brush charged with Punic wax melted and tempered with oil: then, with live coals in an iron pan, the wall should be thoroughly heated, so as to melt the wax and make it lie even, and then rubbed with a candle and clean cloth, as they do marble statues. The coat of Punic wax prevents the effect of the Moon’s as well as that of the Sun’s rays thereon which injure and destroy the colours in work of this nature.”
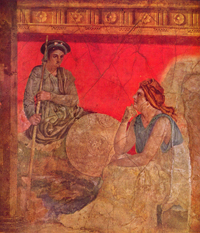
damagedue to blackening of
cinnabar in
the Poppea’s villa in Oplonti
Image courtesy of Mario Pagano
Despite the Punic wax, ancient Pompeian walls have lost their colours in recent years. The most common explanation is that the exposure to the Sun transforms cinnabar into another phase (in which the same atoms, mercury and sulphur, are arranged differently), metacinnabar, which is black. However, the causes and mechanisms have remained a mystery and conservators have been losing a race against time to prevent the degradation – until now.
New results
A Franco-Italian team of researchers studied four samples of wall paint from Villa Sora using the European Synchrotron Radiation Facility (ESRF) in Grenoble, France, to monitor the chemical processes taking place in the walls. The samples of wall painting were taken from both altered and unaltered areas and submitted to microanalyses to shed light on this dramatic blackening.
The team identified the elements present in both well preserved (red) and altered (grey-black) samples. In addition to the sulphur and mercury originating from the pigment, and the calcium present as calcite in the mortar, they detected low levels of other elements, including aluminium, silicon and potassium. However, the most interesting element found was chlorine, which could provide answers to the mysterious blackening process.
By mapping micro X-ray fluorescence signals (see box) over red, grey or black surfaces, scientists were able to directly correlate the distribution of elements to the visible appearance of paintings. This technique is often used on paintings, but normally employs electron microscopy rather than the more sensitive synchrotron analysis. The synchrotron elemental mapping of one of the samples showed that the chlorine distribution perfectly matched a grey debased patch.
Chlorine was found to be associated with sodium and mercury, showing that chlorine has reacted with the pigment and that common salt (NaCl) was involved in the degradation process.
Chlorine is known to cause cinnabar to darken in the presence of light. Researchers speculated that the chlorine in the paintings could originate from two sources of salt. First, as Pompeii and Torre del Greco lie by the sea, the paintings were likely to have absorbed salt from the air. Ironically, the second source of salt could be the ‘protective’ Punic wax, which according to Pliny the Elder was made using seawater.
What does it all mean?
So far, the ESRF findings had confirmed the traditional explanation: that the red cinnabar was transformed to the black metacinnabar. Analyses of another, darker, part of the painting, however, revealed a high accumulation of sulphur in areas where chlorine was not present. The speciation, or chemical environment, of the sulphur was investigated to discover what the surrounding atoms were. This was important, because although cinnabar and metacinnabar have the same composition (Hg and S), they can be distinguished by their different atom arrangements. This analysis refuted the hypothesis that cinnabar had undergone a phase transformation into metacinnabar: no metacinnabar was detected at all.
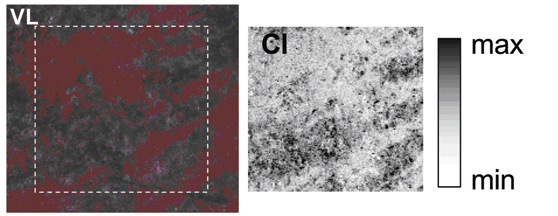
Reprinted with permission from Cotte et al., 2006. Copyright 2007 American Chemical Society
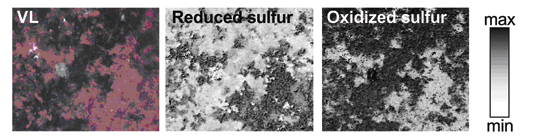
Reprinted with permission from Cotte et al., 2006. Copyright 2007 American Chemical Society
To the scientists’ surprise, they found another compound instead: calcium sulphate, also known as gypsum. This was the sulphurous compound found in the black regions where there was no chlorine. So, instead of a simple phase transition, sulphur had been subjected to a real oxidation, transforming from a reduced sulphide (S(-II)) to an oxidized sulphate (S(+VI)) state. Most probably, gypsum (CaSO4.2H2O) resulted from the reaction of sulphur dioxide (SO2) in the air with the calcite (CaCO3) in the mortar:
SO2(g) + CaCO3(s) + 2H20(g) + ½ O2 ? CaSO4.2H20(s) + CO2
This explanation is supported by the fact that chlorine can catalyse the linkage reaction
HgS ? Hg + S
by which cinnabar dissociates into mercury and sulphur in the presence of light, providing a source of sulphur for further reactions with oxygen in the air and the subsequent formation of SO2. Thus although the second, darker, patch contained sulphur without chlorine, the chlorine in other areas (such as the first, grey, patch) had been sufficient to generate sulphur dioxide which degraded the pigment in other areas.
Thus the synchrotron analyses cast doubt on the traditional theory, that the red cinnabar pigment degraded directly into metacinnabar in the presence of light, and supported the more recent theory that cinnabar dissociates in the presence of chlorine to produce sulphur, which then (in the form of sulphur dioxide), reacts with the calcite in the mortar to form calcium sulphate. This reaction is favoured by the presence of HgS in the pigment, explaining why areas that were not painted with red cinnabar pigment were not discoloured.
Cross-sectional studies
The scientists looked further and investigated the cross-section of one of the samples to map the depth of alteration in the painting. A sample was embedded in resin and polished perpendicularly to the painted layer. In this way, all the layers of the painting, from the mortar to the surface, were accessible. The scientists realised that the degraded layer, containing oxidised sulphur species and sulphates, was only around 5 µ thick and that beneath the surface, the cinnabar had remained intact.
These ancient paintings were frescoes, in which the paint was applied to wet plaster, allowing the pigment to sink in, giving a pigment layer of around 100 µ. The cross-sectional study, therefore, provides two types of information. First, it made it possible to distinguish those elements present in the original painting (calcium, mercury and sulphur) and those which came from the environment (potassium, silicon, aluminium, chlorine and gypsum). This was important, as gypsum itself was sometimes used in the mortar; as it was detected only in the surface layers, it had clearly not been used in the mortar for these paintings. Second, the cross-sections showed that the colour degradation was limited to a very superficial layer, offering hope that the paintings can be restored to their full, colourful glory.
“The research carried out at the ESRF has an extraordinary importance not only for conservation of wall paintings of Villa Sora, but in general for preservation of Roman wall paintings discovered in the most important Roman archaeological sites [such as Pompeii and Herculaneum],” explains Corrado Gratziu, a member of the research team, and a professor emeritus in geology at the University of Pisa, Italy, who specialises in petrology of sedimentary rocks.
Future research
Despite having refuted the classical explanation of cinnabar blackening, the research into the ancient red pigment is far from finished. The complex pathway involving the reaction of both chlorine and sulphur dioxide has raised new questions. “The chemical distribution of the samples is not uniform, which means that atmospheric conditions probably play a role in this change of colours,” explains Marine Cotte, one of the researchers. “The Sun surely influences this process, but the rain may possibly do too,” she adds. “Sunlight accelerates some reactions, while rain can wash the painting and dissolve the more soluble compounds.” Atmospheric contamination or bacterial activities may also contribute to sulphation mechanisms.
The next step for the team is to examine more samples, not only from frescoes in the archaeological site but also from those already excavated and on display in museums. “In this way, we have more data and can compare the results between paintings exposed to different atmospheric conditions and better establish the causes for their degradation,” explains Marine.
X-rays as a tool to study Pompeii
The experiments performed at the ESRF needed a very small (from 100 μm to less than 1 μm) and intense beam of X-rays to detect low concentrations of elements and to provide detailed chemical information. They were performed on the X-ray microscopy beamline (ID21) by combining the techniques of micro X-ray fluorescence mapping and micro X-ray absorption spectroscopy. The former was used to detect the presence of chlorine and sulphur, the latter to identify their speciation, i.e. the way they are bound to other atoms. Below are simple explanations of X-ray fluorescence and spectroscopy, which may be suitable for younger school students.
Principle of X-ray fluorescence
A simple way to understand the principle of X-ray fluorescence is to consider that atoms (the small elements that compose matter) are like houses, with occupants which are named electrons. These occupants are quite lazy, and do not like to live on high floors. So, they will first occupy the ground floor; when there is no more room there, they will occupy the first floor, and so on and so forth. As in life, there are small houses (small atoms, with few electrons) and big houses (big atoms, with many electrons).
The principle of X-ray fluorescence is to shine X-ray light on these atoms. This light will behave like a lift in the house that transfers the electrons living on low floors to floors higher up the house; this process is called ‘absorption’, as light is absorbed by the atom (the electron is absorbed by the lift). As these electrons depart, some empty space will be available on these low levels. Consequently, electrons living higher are quite happy to move downstairs. During this transition, they will express their happiness by emitting some light, or fluorescence. In a small house, the transition will be small, so the shout of joy will be small, whereas in a big house, some transitions – and the corresponding shouts of joy – may be large. By measuring the fluorescence signal (the energy of fluorescence, which is the distance between the departure and arrival floors), one can distinguish small and big atoms, and more precisely, identify each atom according to its characteristic shout of joy.
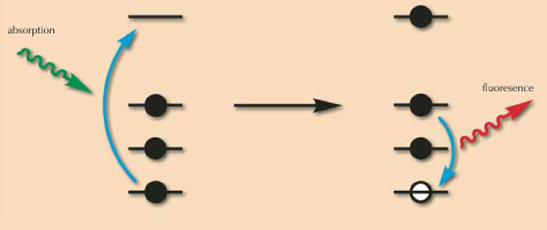
Image courtesy of Calvero; image source: Wikimedia Commons
Principle of X-ray spectroscopy
This method is based on the variation of the X-ray energy in the light needed to excite the atoms, which corresponds to the height of the lift. Let us imagine that the atom is illuminated with a low energy, i.e. that the house has a small lift that goes only from the ground floor to the first floor. If both these floors are full, no electron from the ground floor will take the lift as there is no free space for it on the first floor. There is thus a condition that must be satisfied before an electron can take the lift (or an atom can absorb light): the lift height must be sufficiently large to enable the transition from low floors to higher, empty floors. For example, a lift that is higher than the height of the house will always lead to absorption.
There is a critical case where the lift is just sufficient to lead electrons to the garret, or attic. In fact, electrons prefer to go to the garret rather than being completely thrown out of the house. So the probability of an electron taking the lift to the garret is higher than the probability of it going outside. By measuring the absorbance, we measure the probability that an electron takes the lift. For each lift height (X-ray energy), the absorbance is measured. The reason that scientists are interested in electrons travelling to the garret (being excited to the highest energy level without leaving the atom) is that the height of the garret tells us about the chemical environment of the atom.
In other words, let us consider a lonely house. We can identify this house using fluorescence. Now, let us suppose that this house is surrounded by other houses (atoms). Some connections will be created between them, such as footbridges, on top of the houses. To accommodate a safe footbridge, the house will be slightly rebuilt, changing the level of the garret somewhat in each house. The result is that the garret height depends on the surrounding houses (which houses are in the neighbouring area, how far away they are, and so forth). By measuring the height of the lift to the garret, we can deduce the nature of the footbridge, and consequently the chemical environment of the house (what other atoms surround it, for example). This process is called atomic speciation.
Resources
- Cotte M, Susini J, Metrich N, Moscato A, Gratziu C, Bertagnini A, Pagano M (2006) Blackening of Pompeian cinnabar paintings: X-ray microspectroscopy analysis. Analytical Chemistry 78: 7484-7492. doi:10.1021/ac0612224
Institutions
Review
This article is particularly suitable for teachers looking for new ideas to show their pupils the importance of chemistry and physics in different fields (e.g. archaeology, heritage conservation,…) and for secondary school students fascinated by the study of antiquity. In a plain style enriched by precise chemical information and illuminating metaphors on electrons’ behaviour, the reader can discover how modern investigators solve mysteries about 2000–year-old materials and technologies.
Recovering Pompeii can be used in the classroom to address different science topics (chemistry: atomic and molecular structure, redox, catalysis; physics: atomic structure, electromagnetic radiation; earth science: meteorology, volcanism) as warm-up activity to raise interest in the applications of scientific research. It can be exploited as background reading before a visit to an archaeological site or to research facility like a synchrotron radiation plant. The article is also suitable to test students’ comprehension of language and scientific content.
For example, the following questions could be posed:
- The colours of the studied frescoes were:
- red, grey and green
- red, brown and grey
- red, grey and black
- red, black and brown
- Which of the following compounds does not contain sulphur?
- cinnabar
- calcite
- metacinnabar
- gypsum
- Choose the correct statement about the study of frescoes by means of X-rays:
- X-ray fluorescence measures light absorption and X- rays spectroscopy measures emission
- X-ray fluorescence measures light emission and X- rays spectroscopy measures absorption
- both X-ray fluorescence and X- rays spectroscopy measure light emission
- both X-ray fluorescence and X- rays spectroscopy measure light absorption
Teachers could use the article to discuss;
- the role of chemistry and physics in different fields (research, industry, heritage conservation,…)
- the importance of team work in research as well as in other situations
- the interdisciplinary approach of modern archaeology.
Finally this material could be very useful to start an interdisciplinary project linking science and humanities, to help students understand the underlying unity of culture.
Giulia Realdon, Italy