More than meets the eye: the exotic, high-energy Universe Understand article
In the third article in this series on astronomy and the electromagnetic spectrum, learn about the exotic and powerful cosmic phenomena that astronomers investigate with X-ray and gamma-ray observatories, including the European Space Agency’s XMM-Newton and INTEGRAL missions.
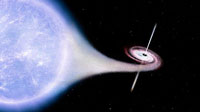
Medialab
In the 1960s, the advent of the space age initiated the era of high-energy astronomy. For the first time, astronomers could see the Universe with X-ray and gamma-ray eyes. Electromagnetic (EM) radiation at these wavelengths is emitted by cosmic sources with extreme properties such as exceptionally high temperatures, extraordinarily high densities or remarkably strong magnetic fields. Ground-based observatories, however, had been unable to register these rays, which have wavelengths too short to penetrate Earth’s atmosphere (figure 1). It took the first space observatories to unveil this turbulent and ever-changing Universe.
In just half a century, observations made at the highest energies have significantly changed our view of the cosmos. By studying the X-ray and gamma-ray sky, astronomers have discovered several new types of astronomical sources and have enhanced their knowledge of many other types of objects. To examine the Universe in the X- and gamma-ray range of the EM spectrumw1, the European Space Agency (ESA; see box) operates two missions: the XMM-Newton (X-rays) and INTEGRAL (X-rays and gamma rays) space observatories. The techniques used in X-ray and gamma-ray astronomy and by these two missions were introduced in the second article in this series (Mignone & Barnes, 2011b); this article provides an overview of what these missions have taught us, from the life of stars to the structure of the Universe. For an overview of the EM spectrum and its role in astronomy, see the first article in this series (Mignone & Barnes, 2011a).
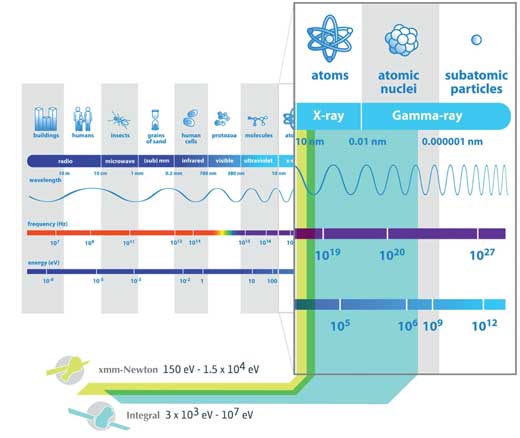
Click on image to enlarge
Image courtesy of ESA/ AOES Medialab
Unveiling the birth and death of stars
Stars are born when gravity causes huge clouds of gas and dust to collapse, fragment and form protostars. These protostars later grow into fully fledged stars when nuclear fusion ignites in their cores. How a star then continues to evolve depends on its mass, with massive stars destined to a shorter life and a more spectacular demise than their lower-mass counterparts (figure 2).
It is the early and late stages of a star’s life cycle that are the most interesting for X-ray and gamma-ray astronomers. Because some very young stars shine brightly under X-rays, astronomers can detect many of them by looking at star-forming regions with X-ray telescopes such as XMM-Newton (figure 3). The most massive young stars release highly energetic radiation and extremely hot gas, which are observed at X-ray wavelengths and influence how other stars form in the surrounding area. Astronomers using XMM-Newton have detected bubbles of hot gas from young massive stars in many regions of the skyw2, including the Orion Nebula and the star-forming region NGC 346. This research feeds into our understanding of how young massive stars affect star formation around them – a hot topic in modern astrophysics.
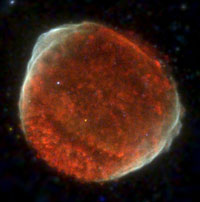
supernova remnant SN 1006
as viewed with XMM-
Newton. This object is the
remains of a supernova that
was seen by Chinese
astronomers in 1006 AD.
Visible in the upper-left
and lower-right corners are
shock waves where particles
such as electrons are
accelerated to very high
speeds
Image courtesy of CEA / DSM
/ DAPNIA / SAp / J Ballet and
ESA
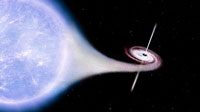
of an X-ray binary. With its
intense gravitational field the
black hole on the right draws
matter from its companion, a
blue super- giant star, on the
left. The stripped material
spirals around the black hole,
forming an accretion disc,
which shines brightly at the
highest energies. Two
powerful jets of highly
energetic particles stem from
the vicinity of the black hole
Image courtesy of ESA / AOES
Medialab
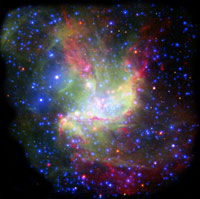
region NGC 346 is located in
the Small Magellanic Cloud,
one of the Milky Way’s
neighbouring galaxies. This
false-colour image combines
observations performed with
XMM-Newton in X-rays
(blue) with data gathered in
visible (green) and infrared
(red) light with the Hubble
and Spitzer space telescopes,
respectively
Image courtesy of NASA / JPL-
Caltech / D Gouliermis (Max-
Planck Institute for Astronomy,
Heidelberg, Germany) and ESA
At the ends of their lives, massive stars explode as supernovae (as described in Székely & Benedekfi, 2007), heating the surrounding gas to extremely high temperatures and accelerating particles, such as electrons, to very high speeds. As a result, an abundance of X-rays and gamma rays are released (figure 4). Furthermore, many elements heavier than iron, such as lead, nickel and gold, are synthesised during supernova explosions (to learn more, see Rebusco et al., 2007). Some of these elements are radioactive and eventually decay into stable isotopes, producing gamma rays in the process. Astronomers using INTEGRAL have surveyed the Milky Way and found traces of the radioactive isotope aluminium-26. Just like archaeologists, they have delved into the history of our galaxy and performed a census of past supernovae. The results demonstrate that, in the Milky Way, supernovae occur on average once every 50 yearsw3.
After a supernova explosion, all that remains of the massive star is an extremely compact and dense object – either a neutron star or a black hole.
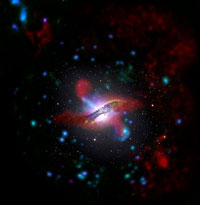
galaxy, Centaurus A (NGC
5128). This false-colour
image combines observations
performed with XMM –
Newton in X-rays (cyan, blue
and purple, in order of
increasing energy) and
data gathered at longer,
far- infrared (yellow) and
sub- millimetre (red)
wavelengths using ESA’s
Herschel Space Observatory.
At X-ray wavelengths, a
number of foreground point
-like sources are visible:
these are X-ray binaries
belonging to our galaxy, the
Milky Way
Image courtesy of ESA / XMM-
Newton (X-rays); ESA /
Herschel / PACS / SPIRE / CD
Wilson, McMaster University,
Hamilton, Ontario, Canada (far
-infrared and sub-millimetre)
With such a huge mass squeezed into a restricted space, these remnants have exceptionally strong gravitational fields and exert an intense pull on nearby matter, but they are fairly difficult to detect. However, if the neutron star or black hole is part of a binary stellar system (two stars orbiting around a common centre of mass), it may start devouring matter from its companion star; the accreting matter then heats up to millions of degrees, emitting X-rays and gamma rays. This high-energy emission can be used to reveal the presence of a neutron star or black hole.
These systems are called X-ray binaries (figure 5) and were discovered in the late 1960s via X-ray observations. Back then, neutron stars and black holes had only been predicted by theory, so these observations provided the first proof of their existence.
Since then, several generations of space-based observatories have helped astronomers to learn more. XMM-Newton and INTEGRAL have studied many X-ray binaries (which may also release gamma rays), revealing important details about the physics of black holes and neutron stars. For example, gammarays from Cygnus X-1, observed using INTEGRALw4, helped astronomers to better understand how matter is accreted via a disc onto this black hole and partly expelled in two symmetric jets.
The distant Universe
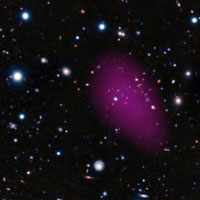
very distant galaxy cluster CL
J1449+0856 performed in X-
rays (purple glow) with
XMM- Newton are
superimposed onto an image
taken with ground-based
telescopes at near-infrared
wavelengths. Most objects
visible in the image are very
faint and distant galaxies.
The galaxies belonging to the
galaxy cluster are visible as
a clump of faint, red objects.
With a temperature above 20
million Kelvin, the hot gas
pervading the intergalactic
space shines brightly in
X-rays
Image courtesy of ESA / ESO /
Subaru / R Gobat et al.
High-energy astronomers not only observe the birth and death of stars within the Milky Way and nearby galaxies, but also use X-rays and gamma-rays to investigate the much more distant Universe – including super-massive black holes and clusters of galaxies.
All large galaxies harbour super-massive black holes at their cores, with masses a few million to a few billion times that of the Sun. Some galaxies, known as active galaxies, contain super-massive black holes that, unlike the one in the centre of the Milky Way, are active. Devouring matter from their surroundings, these black holes release high-energy radiation as well as powerful jets of highly energetic particles (figure 6).
ESA’s XMM-Newton and INTEGRAL are thus ideal tools to hunt for active galaxies and to investigate the mechanisms that power them. Astronomers cannot see all the necessary details in more distant high-energy sources, so they also collect data from as many nearby active galaxies as possible. By combining data from close and distant galaxies, astronomers have figured out how super-massive black holes accrete matter via a disc, and how these discs may be surrounded by absorbing clouds of gasw5.
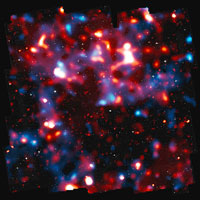
the distribution of ‘normal’
matter, traced via hot gas
seen by XMM-Newton (in
red) and stars and galaxies
observed with the Hubble
Space Telescope (in grey), to
the distribution of the
invisible dark matter (in
blue), which has been
inferred from the
gravitational lensing effect.
The map demonstrates how
‘normal’ matter across the
Universe follows the
structure of an underlying
‘scaffolding’ of dark matter
Image courtesy of NASA / ESA /
R Massey (California Institute of
Technology)
On a still larger scale, galaxies tend to assemble in clusters of up to several thousand galaxies. These clusters are the largest structures in the Universe to be held together by gravity, and release a diffuse X-ray glow. This glow, first observed in the 1970s, revealed that the intergalactic space in a cluster contains an enormous amount of hot gas. Together with other observatories that probe the sky across the EM spectrum, XMM-Newton has observed hundreds of galaxy clusters (figure 7).
These include a very distant cluster that is one of the earliest structures to have formed in the Universew6, just 3 billion years after the Big Bang. This may sound like a very long time, but it is less than one quarter of the Universe’s present age.
Galaxy clusters are located in the densest knots of the cosmic web, the gigantic network of structure that makes up the Universe and consists mostly of invisible dark matterw7. Using XMM-Newton, astronomers have spotted matter where it is most densely concentrated, thus tracing the distribution of cosmic structure across the Universe (figure 8).
From the birth of a star to the structure of the Universe – what next? X-ray and gamma-ray observatories, including ESA’s XMM-Newton and INTEGRAL, continue to keep a close watch on the ever-changing, high-energy sky, recording sudden violent outbursts of X-rays and gamma-rays. By continuing to unveil celestial wonders to astronomers, these remarkable space observatories are helping to solve the mysteries of our Universe.
More about ESA
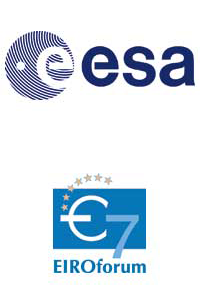
The European Space Agency (ESA)w8 is Europe’s gateway to space, organising programmes to find out more about Earth, its immediate space environment, our Solar System and the Universe, as well as to co-operate in the human exploration of space, to develop satellite-based technologies and services, and to promote European industries.
The Directorate of Science and Robotic Exploration is devoted to ESA’s space science programme and to the robotic exploration of the Solar System. In the quest to understand the Universe, the stars and planets and the origins of life itself, ESA space science satellites peer into the depths of the cosmos and look at the furthest galaxies, study the Sun in unprecedented detail, and explore our planetary neighbours.
ESA is a member of EIROforumw9, the publisher of Science in School.
References
- Mignone C, Barnes R (2011a) More than meets the eye: the electromagnetic spectrum. Science in School 20: 51-59.
- Mignone C, Barnes R (2011b) More than meets the eye: unravelling the cosmos at the highest energies. Science in School 21: 57-64.
- Rebusco P, Boffin H, Pierce-Price D (2007) Fusion in the Universe: where your jewellery comes from. Science in School 5: 52-56.
- Székely P, Benedekfi Ö (2007) Fusion in the Universe: when a giant star dies….Science in School 6: 64-68.
Web References
- w1 – To learn more about X-ray and gamma-ray astronomy at ESA, watch Rebecca Barnes in Episode 5 of the Science@ESA vodcast: The untamed, violent Universe.
- w2 – Find out more about how XMM-Newton helped in studying the star-forming region NGC 346 and detected a hot-gas bubble in the Orion Nebula.
- w3 – Learn how INTEGRAL identified the supernova rate for the Milky Way and how XMM-Newton helped to analyse the remnants of the Tycho supernova.
- w4 – The ESA website has more information about X-ray binaries.
- Find out how gamma rays from the Cygnus X-1 jets were observed with INTEGRAL (‘INTEGRAL spots matter a millisecond from doom’).
- Learn about how Supergiant Fast X-ray Transients were observed by XMM-Newton (‘Neutron star caught feasting on clump of stellar matter’).
- w5 – Read more details of ESA’s findings about active galaxies with XMM-Newton and INTEGRAL.
- w6 – Read about how XMM-Newton helped discover an old galaxy cluster in the young Universe.
- w7 – Learn about the first 3D map of the Universe’s dark matter scaffolding.
- w8 – For more information on the European Space Agency, visit the ESA website.
- w9 – Find out more about EIROforum.
Resources
- ESA has produced many more education materials.
- All education materials produced by ESA are freely available to teachers in the 18 ESA member states. Many are translated into several European languages.
- Learn more about the activities of the European Space Agency’s Directorate for Science and Robotic Exploration.
Institutions
Review
This article, the third in a series, describes European research activities within the field of high-energy astronomy. The second article in the series described the techniques used by two ESA missions, XMM-Newton (X-rays) and INTEGRAL (X-rays and gamma rays); this article describes some of their results, including insights into the birth and death of stars, as well as the more distant Universe.
For older students (16+), the article is ideal for physics lessons, where it could be used to address astrophysics (the life of stars, cosmic objects, the Big Bang theory), optics or even quantum physics (spectral ranges, relationship between wavelength and energy, EM waves), mass and gravity. It could also be used in geography lessons about the Universe, solar systems and cosmic objects.
To make it accessible to younger students (age of 10-15 years) as well, I would suggest the teacher selects just parts of the article to discuss.
The article could be very useful in English lessons too, or – once it has been translated – in German, French or other language lessons. Because the article is not too technical, even teachers who are not very familiar with physics could use it.
The article could also be used to stimulate discussion, with questions including:
- Describe the European Space missions XMM-Newton and INEGRAL.
- Give an overview of the electromagnetic spectrum (including visible, infrared and UV).
- What is the relationship between wavelength, energy and frequency?
- Why do we use space observatories in addition to ground-based observatories?
- Why are the sources that shine most brightly in gamma rays hotter than those that shine most brightly in X-rays?
- What are X-ray binaries?
- What can happen to massive stars at the ends of their lives?
Gerd Vogt, Higher Secondary School for Environment and Economics, Yspertal, Austria