Fuelling interest: climate change experiments Teach article
Dudley Shallcross, Tim Harrison, Steve Henshaw and Linda Sellou offer chemistry and physics experiments harnessing alternative energy sources, such as non-fossil fuels.
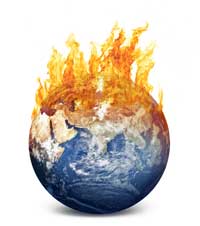
iStockphoto; earth image
courtesy of NASA
Discussions of climate change in the science classroom can be very wide-ranging, but will probably involve different sources of energy and their consequences. Relevant topics are likely to include different fuels that can be used, how effective they are and how they are produced, and alternatives to combustion. We suggest a couple of laboratory activities to support physics and chemistry lessons on climate change.
1) Measuring fuel efficiency
Unburned fuel evaporating into the atmosphere, during either storage or combustion, is an air pollutant, and also plays a role in determining the lifetime of greenhouse gases. One way to limit the damage is to use alcohols instead of more standard hydrocarbon fuels such as petrol and diesel.
Hydrocarbons in the atmosphere are oxidised by the hydroxyl radical (·OH) to form predominantly alcohols and carbonyls. Levels of atmospheric ·OH are important, as these radicals remove greenhouse gases that contain the C-H bond, such as CH4. Computer simulations have shown that releasing alcohols into the atmosphere has a less damaging effect than releasing hydrocarbons, because fewer oxidation steps take place, removing smaller amounts of ·OH. Therefore, using alcohols instead of hydrocarbons as fuels has a positive effect on both air quality and the removal of greenhouse gases. Moreover, the smaller alcohols released into the atmosphere can be removed, to a small extent, by physical processes such as dry (taken up by a surface) and wet (rain, fogs, aerosols) deposition, whereas their hydrocarbon counterparts cannot.
Alcohols are used in fuel cells (see ‘Fuel cells: energy from electrolysis’) which have a wide range of potential applications, for example in short-range vehicles.
Of course, a good fuel is not only environmentally friendly – it must also be an efficient source of energy. The following experiment allows students to determine the energy released by burning different types of alcohols, and to compare their effectiveness with that of more standard fuels.
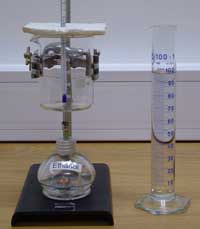
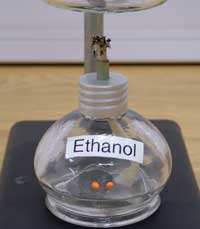
Images courtesy of Bristol ChemLabS
Alcohol burners are the small burners made of glass, complete with a wick, which usually come with children’s chemistry sets and are readily available from schools equipment companies. They can be used to determine the energy released in the combustion of shorter primary alcohols such as methanol, ethanol, propan-1-ol, butan-1-ol and pentan-1-ol.
The students can set up the experiment (see images above), in which the alcohol burner is filled with a known alcohol. First, weigh the burner and its contents. Measure out 150 ml of water accurately and place it in a 250 ml glass beaker. We assume that 1.0 ml of water weighs 1.0 g. Measure the water temperature at the beginning of the experiment and clamp the beaker above the burner, leaving a space of about 5 cm between the wick and the base of the beaker. Ignite the burner and place it centrally under the water container until the temperature has risen by 30 to 40 °C. Determine the temperature rise by recording the final temperature of the water. Weigh the final mass of the alcohol burner and its contents. It is at this stage that the students will not remember if they originally weighed the alcohol burner with the lid on or off! Calculate the mass of alcohol burned (MB).
The energy released in the combustion, used to raise the water temperature, can be calculated using equation (1)
ΔHC = -c x Mwater x TR (1)
where:
ΔHC = heat of combustion [kJ]
c = the specific heat capacity of water = 4.187 kJ kg-1 °C-1
Mwater = mass of water [kg]
TR = temperature rise of water [°C]
The combustion energy can then be expressed as energy per gram or energy per mole of the alcohol burnt, in order to compare the results obtained by several groups with the same alcohol or with different alcohols.
Energy of combustion per gram = ΔHC / MB (2)
Energy of combustion per mole = ΔHC x Mr / MB (3)
where:
Mr is the relative molecular mass of the alcohol being burnt,
MB is the mass of the burnt alcohol.
Further investigations that can be carried out by the students include:
- Change the alcohol that the burner uses. In practice, it is best to have sets of alcohol burners that use different alcohols, as it is very difficult to replace the alcohol in a wet wick
- An alternative to comparing several different alcohols is to compare just one alcohol with a night light (a small wax candle in a metal holder)
- Change the material that the beaker is made from, for example to copper or a galvanised steel food can
- Change the thickness of the material that the water container is made from
- Compare an insulated container (made from a heatproof material!) with a glass beaker
- Compare the open glass beaker being heated to one with a lid fitted on top, through which the thermometer is placed.
- Does it make a difference whether the water in the container is stirred or not?
- Does the height at which the beaker is clamped above the flame make any difference to the amount of heat absorbed by the water?
- Does it make a difference to have boards / heatproof mats placed around the burner to reduce draughts?
- Does the length of wick that is exposed make any difference?
Note that it is too dangerous to use petrol or diesel in these burners. Even without testing conventional fuels or different alcohols to compare the energies released by ‘green’ fuels, these experiments can be used to discuss experimental error and accuracy of measurement in general. It will be obvious from comparing the classroom experimental results with the textbook data or those available on Wikipediaw1 (see table) that there are significant errors.
Name of alcohol / fuel | Standard heat of combustion [MJ / kg] |
---|---|
Peat (damp) | 6.0 |
Peat (dry) | 15.0 |
Wood | 15.0 |
Coal (Lignite) | 15.0 |
Methanol | 22.7 |
Coal (Anthracite) | 27.0 |
Ethanol | 29.7 |
Carbon | 32.8 |
Propan-1-ol | 33.6 |
Butan-1-ol | 36.2 |
Pentan-1-ol | 37.7 |
Diesel | 44.8 |
Paraffin | 46.0 |
Kerosene | 46.2 |
Gasoline | 47.3 |
Butane | 49.5 |
Propane | 50.4 |
Ethane | 51.9 |
Natural gas (average, varies depending on source country) | 54.0 |
Methane | 55.5 |
Hydrogen | 141.8 |
2) Producing biofuels from vegetable oil
Biofuels are solid, liquid or gaseous fuels derived from relatively recently dead biological material, as opposed to fossil fuels, which are derived from long-dead biological material.
Whereas burning fossil fuels releases CO2 which has been trapped for a long time, burning biofuels should only release CO2 that has been recently captured from the atmosphere during photosynthesis and converted to fuel. In theory, the process should therefore be carbon neutral. The production of biofuels, however, also generates CO2; the life-cycle emission (amount of CO2 generated during production, use and waste disposal) of some first-generation biofuels even exceeds that of traditional fossil fuels.
So far, three generations of biofuels have been developed: first-generation biofuels are made from sugar, starch, vegetable oil, or animal fats. Besides having sub-optimal emission levels, first-generation biofuels have other important limitations: there is a threshold above which their production threatens food supplies and biodiversity. Moreover, they are more expensive than existing fossil fuels.
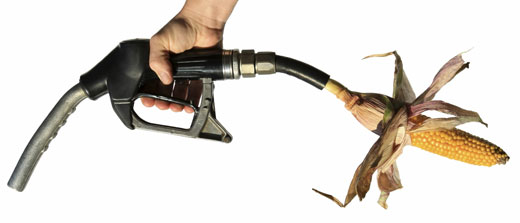
This led to the development of second-generation biofuels, which can supply a larger proportion of our fuel supply sustainably, affordably and with greater environmental benefits. They use a variety of non-food crops, including waste biomass, the stalks of wheat, corn, and wood.
Still, land-based biofuels occupy land that could otherwise be used to grow food. Algae fuel, also called oilgae, is being developed as a third-generation biofuel to avoid this problem. These fuels can be produced in a low-input, high-yield manner, since algae produce 30 times more energy per acre than land crops such as soybeans.
Theoretically, biofuels can be produced from any biological carbon source, although the most common sources are photosynthetic plants and plant-derived materials. One advantage of many biofuels over most other fuel types is that they are biodegradable, and so relatively harmless to the environment if spilled.
There are a number of ways to make biofuels from a range of vegetable oils, but the reaction is essentially the same. A biofuel is made by alkaline hydrolysis of the triglycerides in a vegetable oil (see image above), and the following re-esterification of the triglycerides to the methyl ester. In practice, both steps can take place in the same preparation, provided a mixture of methanol in alkali is used, as is done commercially. This mixture contains the methoxide ion. During hydrolysis, a fatty acid is liberated from the triglyceride. Together with the methoxide ion, the methyl ester of the fatty acid is then formed.
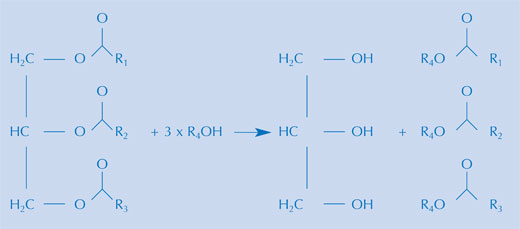
Image courtesy of Bristol ChemLabS
Glycerol (propan-1,2,3-triol) is a waste product of this last reaction. The disposal or use of the glycerol is one of the challenges for this growing industry.
For the experiment described below, you can either use a boiling tube or a reflux method involving QuickfitTM laboratory glassware.
In the simpler preparation without a reflux, 12-13 ml of a vegetable oil of choice are put into a boiling tube with 2 ml of potassium hydroxide in methanol (5% w/w). The liquids are mixed without shaking to prevent trapping air and foaming. The mixture is left to stand in a water bath at 60ºC. The reaction rate can be followed by measuring the viscosity: you can time how long it takes for a small ball bearing (readily available, symmetric shape, smooth and dense) to drop through a defined depth of the mixture in the tube every 5 minutes at regular intervals for up to 2 hours.
The larger triglyceride is broken down to smaller subunits (see image above), which will then have a smaller mass than the compound from which they are formed. Since the viscosity of a liquid is directly proportional to the mass of its molecules, the reaction will lead to a less viscous mixture. Furthermore, you can leave a sample for a whole day in these conditions to qualitatively observe the extent of hydrolysis.
Although the biofuel produced in this experiment still contains the glycerol formed as a waste product of the reaction, the change in viscosity can nevertheless be observed. If using a reflux condenser for the reaction, the biofuel can be separated from the glycerol by solvent extraction.
The biofuel produced by either method can then, for example, be used to run a small diesel engine.
Note: if using vegetable oil that has already been used for cooking, please remember to filter out any food residues first!
3) Fuel cells: energy from electrolysis
Both the previous experiments are based on the release of energy through combustion. While this is one way of oxidising a fuel to release energy, it is not the only way. Fuels can also be oxidised by electrolysis, as demonstrated in the following experiment.
Fuel cells produce electricity from a reaction between a fuel such as an alcohol or hydrogen at the anode, and an oxidising agent such as oxygen or chlorine at the cathode. The fuel and the oxidising agent react in the presence of an electrolyte. The reactants flow into the cell, and the reaction products flow out of it, while the electrolyte remains within it. A fuel cell works by catalysis, separating the component electrons and protons of the reactant fuel, and forcing the electrons to travel through a circuit, hence converting them to electrical power.
A fuel cell is different from a chemical battery because its reactants can be replenished (representing a thermodynamically open system), whereas the chemicals being consumed in a battery are not, as it is sealed (thermodynamically closed). A fuel cell will continue to work as long as its reactants are replaced.
A typical fuel cell produces a voltage from 0.6 V to 0.7 V at full rated load. However, fuel cells can be combined in series and parallel circuits; series yield higher voltage, while parallel allows a stronger current to be drawn. Such a design is called a fuel cell stack. Furthermore, the cell surface area can be increased to allow stronger current from each cell.
Fuel cells are very useful as power sources in remote locations, such as spacecraft, remote weather stations, large parks and rural locations. In the future, they could also power vehicles.
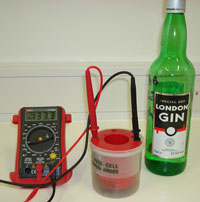
Image courtesy of Bristol ChemLabS
There are several demonstration fuel cells available for purchasew2 (for about €20 each), to show the principle to students. The one we use is an alcohol/air fuel cell (see image to the left), which consists of two parts: an adapted plastic beaker with a conductive pad connected to a terminal (the anode), and an insert containing the catalyst. A defined volume of a source of alcohol (such as antifreeze), or of an alcohol itself (such as propan-1-ol) is mixed with 55 ml of alkali (such as sodium hydroxide) as an electrolyte, and put into the plastic beaker. The insert is put into the beaker to complete the cell. Air can pass through the insert into the alkaline alcohol mixture.
The chemical reaction that generates a current is the oxidation of the alcohol by the oxygen in the air – to a carbonyl or a carboxylic acid, depending on the degree of oxidation. The reaction is catalysed by platinised graphite in a pad at the base of the beaker.
At the cathode: 4e– + O2 + 2H2O → 4OH–
At the anode: CH3CH2OH + 2OH– → CH3CHO + 2H2O + 2e–
The aldehyde CH3CHO is also capable of being oxidised to a carboxylic acid, so it can continue to release an electric current. Incidentally, an alternative fuel for this particular type of fuel cell, which gives a greater voltage than an alcohol, is sodium tetrahydroborate (III) (sodium borohydride, NaBH4). According to the manufacturer, only minute quantities (about 20 mg of NaBH4) are required to run the fuel cell for one hour. Note NaBH4 is toxic, harmful and an irritant.
The voltage produced by the fuel cell can easily be measured using a cheap multimeter.
These are some of the investigations that can be carried out by students using the fuel cells:
- Different types of alcohol may be used, as long as they are water-soluble: these include the alcohol series from methanol to pentan-1-ol, the secondary or tertiary isomers of these alcohols (where they exist) and different sources of alcohol, such as methylated spirits, car windscreen wash, antifreeze or even alcoholic drinks like vodka or gin. What effect does the type of alcohol have on the voltage produced?
- Students can investigate the effect of changing the initial concentration of alkali on the output voltage. They may also analyse whether the type of alkali used, for example sodium versus potassium hydroxide, makes any difference to the voltage produced by the fuel cell.
- Investigating the change in concentration of the alkali or the alcohol while the fuel cell is running can also be interesting.
- The whole setup can be placed in a cooled/heated water bath to change the temperature at which the reaction takes place. By measuring the output current, the effect of temperature on the rate of reaction can be investigated.
Suggestions for further experiments can be found in a couple of books or onlinew3.
Acknowledgement
The authors wish to thank Will Davey at Sheffield University for the basis of the biofuel production experiment.
Web References
- w1 – For heat of combustion tables of various fuels and organic compounds on Wikipedia, see: http://en.wikipedia.org/wiki/Heat_of_combustion#Heat_of_combustion_tables
- w2 – One of many suppliers specialising in fuel cell technology for both industry and education is h-tec: www.h-tec.com
- w3 – h-tec has also published a book on using fuel cells in the classroom:
- Voigt C, Hoeller S, Kueter U (2005) Fuel Cell Technology for Classroom Instruction (Basic Principles, Experiments, Work Sheets). Luebeck, Germany: h-tec
- A collection of safe, inexpensive, educational and fun projects focusing on fuel cell technology is:
- Harper G (2008) 101 Fuel Cell Projects for the Evil Genius. New York, USA: McGraw-Hill Professional
- For further experiments using fuel cells, see: www.ectechnic.co.uk/exps.html
Resources
- Harrison T, Shallcross D, Henshaw S (2006) Detecting CO2 – the hunt for greenhouse-gas emissions. Chemistry Review 15: 27-30
- Pacala S, Socolow R (2004) Stabilisation wedges: solving the climate problem for the next 50 years with current technologies. Science 305: 968-972. doi: 10.1126/science.1100103
- Shallcross D, Harrison T (2008) Climate change modelling in the classroom. Science in School 9: 28-33.
- Shallcross D, Harrison T (2008) Practical demonstrations to augment climate change lessons. Science in School 10: 46-50.