Hunting for exoplanets Understand article
The possibility of worlds beyond our own has fascinated people for millennia. Now technology is bringing these other worlds – or exoplanets – within reach of discovery.
“There are infinite worlds both like and unlike this world of ours.” So said the ancient Greek philosopher and atomist Epicurus in the fourth century BCE. But just as Greek ideas of a heliocentric Solar System were lost in the turmoil of history, Epicurus’s notion of a boundless cosmos was swept aside by the dominant philosophy of Aristotle, who claimed that “there cannot be more worlds than one.”
It was nearly 2000 years before the idea that Earth might not be alone was taken seriously again. In Italy in 1584, at the height of the Renaissance, the philosopher Giordano Bruno proposed that the Universe is infinite and that the stars are distant suns orbited by “innumerable worlds”. About 400 years later, in 1992, came the first confirmed detection of one of Bruno’s innumerable worlds, by radio astronomers Aleksander Wolszczan and Dale Frail (Wolszczan & Frail, 1992). Wolszczan and Frail had discovered a furiously fast-spinning pulsar in the constellation of Virgo. Rotating more than 160 times a second, this tiny collapsed star emits powerful radio beams that sweep across Earth as rapid pulsations. An irregularity in the signal led the astronomers to conclude that “the pulsar is orbited by two or more planet-sized bodies”, which became the first known ‘exoplanets’ – planets external to our own Solar System. Three years later, another exoplanet was found by Michel Mayor and Didier Quelozw1, this time in orbit around a Sun-like star called 51 Pegasi. It was an extreme world – a ‘hot Jupiter’-type planet at least 150 times more massive than Earth and orbiting its sun even more closely than Mercury orbits ours.
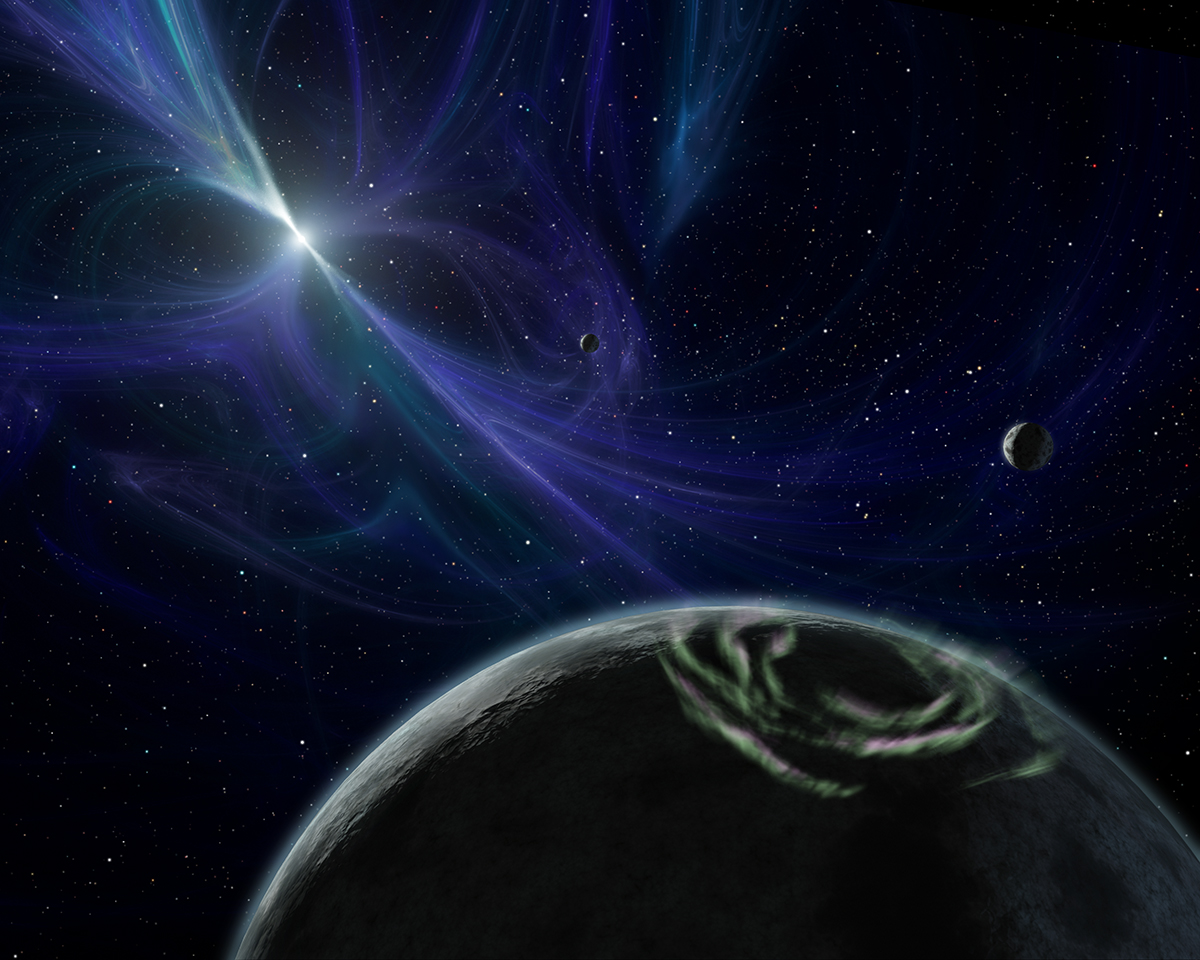
NASA/JPL-Caltech
Astronomers realised it was possible to detect exoplanets, and the race to find more followed. Detection methods improved, and the discoveries piled up.
We now know of more than 4000 exoplanets: most are near our Solar System, as their proximity makes them relatively easy to detect. This suggests that there may be as many as 11 billion Earth-sized and potentially habitable planets in our Milky Way galaxy alone.
Alien worlds
The hunt for exoplanets has been far more successful than astronomers once dared hope. Alien planetary systems are ubiquitous and surprisingly diverse, with many bearing little resemblance to our Solar System. It now appears that most stars have planets, and small rocky planets are abundant, including Earth-like worlds that orbit their parent star in the ‘habitable zone’ where liquid water might exist on the planet’s surface – a condition thought essential for life. Many massive, Jupiter-like exoplanets have also been found, and some have been imaged. We have detected clouds in their skies and, thanks to spectral analysis, we can even identify the elements in their atmospheres.
However, the hunt for exoplanets faces considerable challenges. Planets are much fainter and smaller than the stars they orbit, so we rely mostly on indirect methods to find them, rather than imaging them directly. There are several different approaches, each with its own advantages and disadvantages. In this article, we take a look at some of the most important methods of discovery.
Transit method
If an exoplanet happens to pass in front of its parent star while we watch from Earth – a phenomenon known in astronomy as a ‘transit’ – the star’s brightness will briefly dip, if only by a tiny fraction, as was seen with the triple transit of the star TRAPPIST-1 in 2015 (figure 1). More than three-quarters of known exoplanets were detected this way. The transit method relies on an element of luck: because the inclinations of their orbits are randomly distributed, from our viewpoint most exoplanets are never seen transiting in front of their star. To get around this problem, space telescopes such as NASA’s Kepler telescope survey large numbers of stars over a long period. Stars may also show dips in brightness due to surface features such as star spots, so follow-up observations are needed to confirm a discovery.
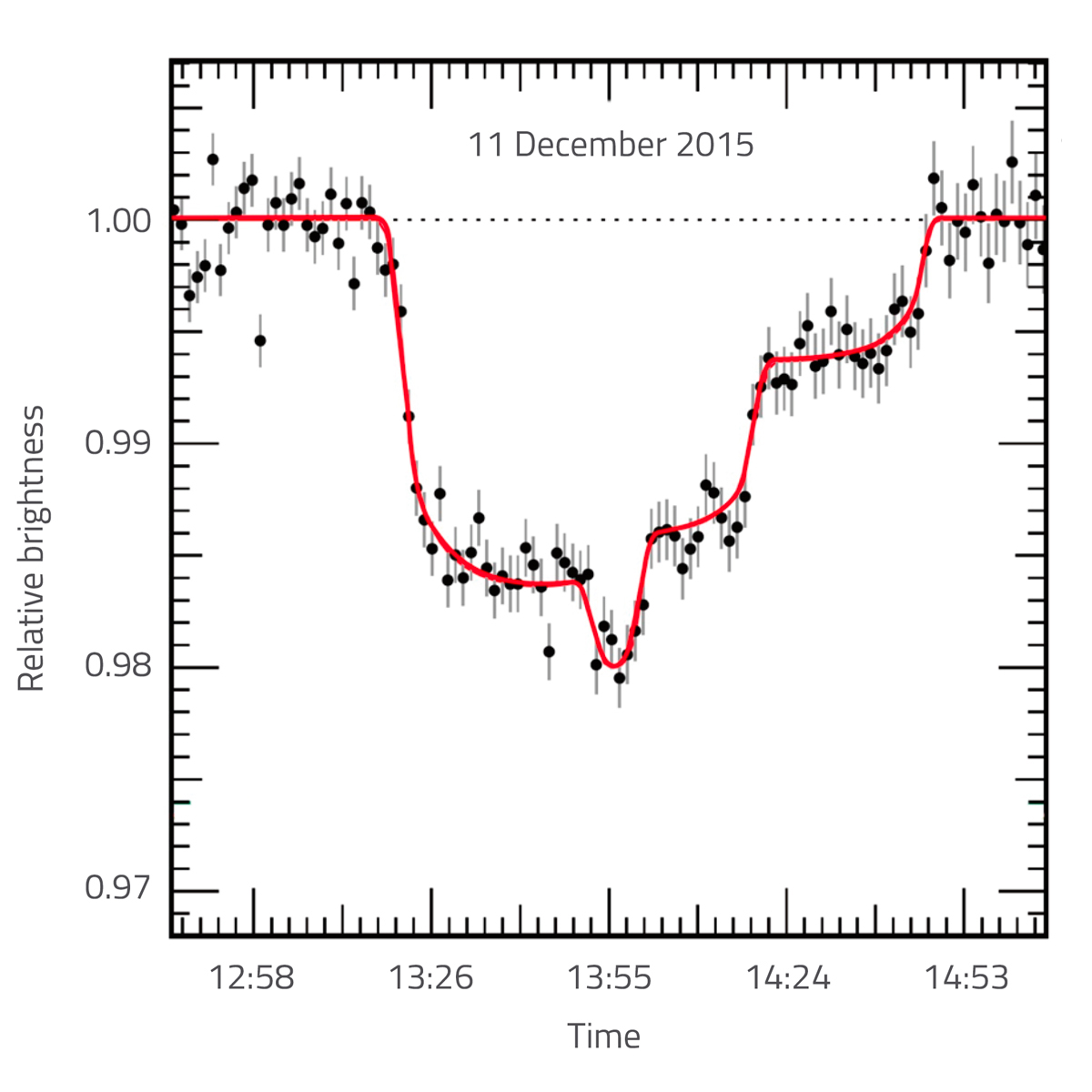
ESO/M Gillon et al., CC BY 4.0
During a transit, the star’s light passes through the exoplanet’s atmosphere, where certain wavelengths are selectively absorbed by elements and molecules in the atmosphere. The pattern of absorption, which we can read by carrying out a spectral analysis, serves as a chemical fingerprint, telling us which substances are present. Such studies have revealed water in exoplanet atmospheres (Tsiaras et al., 2019) and can even tell us whether atmospheric water takes the form of vapour or liquid.
Astrometric method
Planets are far less massive than stars, but they still exert a pull on them. The gravitational tug-of-war between a planet and its star causes the pair to orbit a shared centre of mass that is often within the star itself, although not right in the middle. The result is that the star appears to wobble as it moves in a tiny orbit around the shared centre of mass. The pull of Jupiter on the Sun, for example, causes the Sun to wobble with an average velocity of 12 m/s as it orbits a centre of mass close to its surface. The effect of all the planets in our Solar System on the Sun is the wobbling path shown in figure 2.
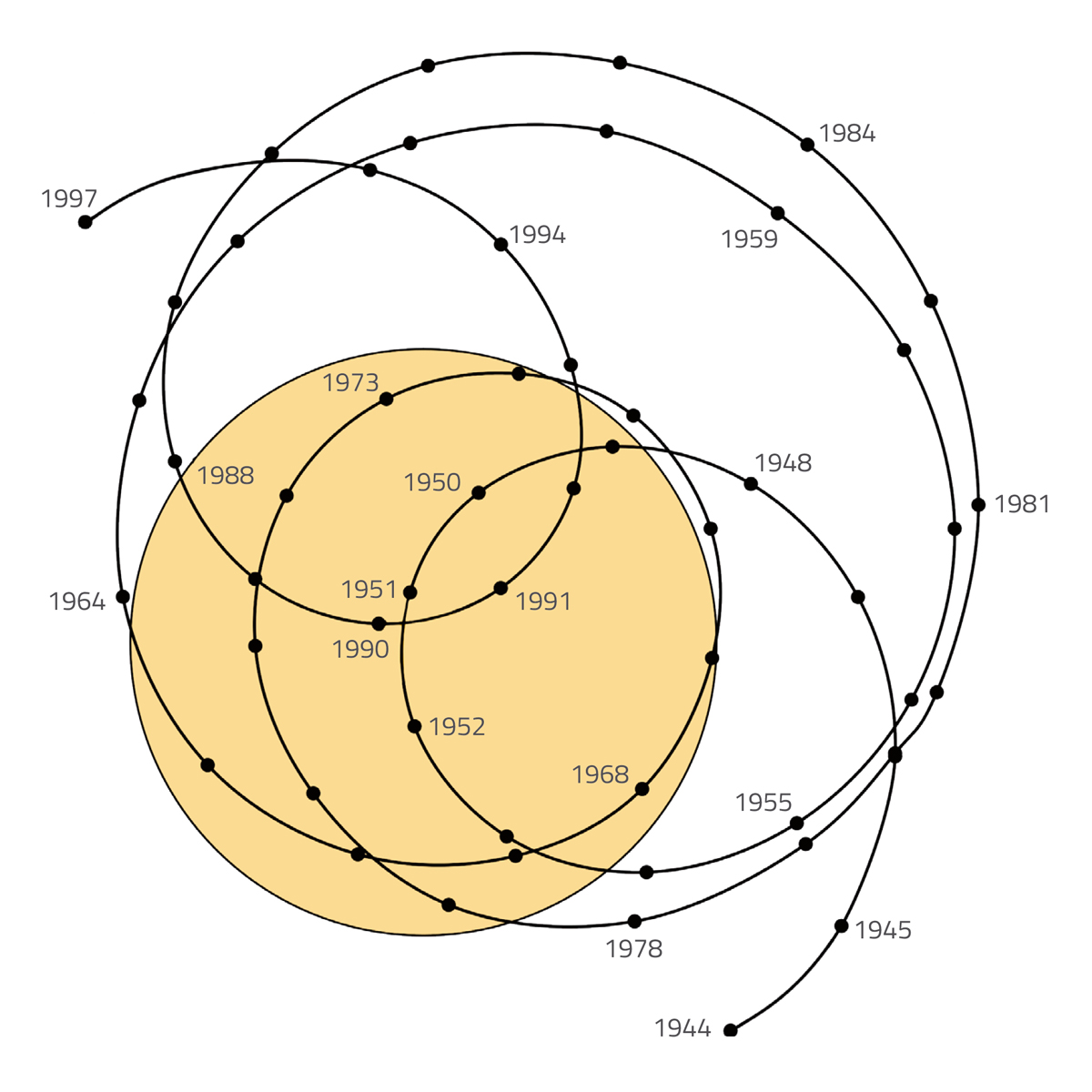
Carl Smith/Rubik-wuerfel/Wikimedia Commons, CC BY-SA 3.0
The astrometric method relies on detecting this telltale but almost imperceptibly small wobble in stars situated many light years away, which places high demands on the sensitivity of instruments. To put this into perspective, a Sun-sized star 42 light years away (ten times further than our closest neighbouring star, Proxima Centauri) would wobble by only a fifth of a millionth of a degree under the influence of a planet like Jupiter. This is equivalent to seeing the International Space Station from the Earth moving 1.5 mm in its orbit. The effect of an Earth-sized planet would be some 1600 times smaller.
Fewer than 0.02% of known exoplanets have been found using this method. However, that figure might rise, thanks to space telescopes such as the one carried by ESA’s Gaia spacecraft. Gaia’s equipment is unaffected by the distortion of Earth’s atmosphere, and can detect movements as small as a quarter of a billionth of a degree.
Radial velocity method
Another way to detect the wobbling motion of a star is to look for shifts in its spectrum. This is called the radial velocity method, and it makes use of the Doppler effect – the compression or stretching of waves from a source that is moving towards or away from the observer. When a star is moving away from us, its light is shifted towards the red end of the spectrum; when it is moving towards us, the light is shifted towards blue.
The shift in wavelength caused by an orbiting planet is tiny. Our Sun’s 12 m/s wobble, caused by Jupiter, shifts its spectral lines by a mere 0.000004%. Even so, astronomical spectroscopes can already detect stellar movements of less than 1 m/s, and work is in progress to reach the 0.1 m/s precision needed to detect Earth-like planets. For this reason, the radial velocity method is a cornerstone of exoplanetary astronomy and accounts for nearly 20% of discoveries made since 2012.
Like other techniques used to hunt for exoplanets, the radial velocity method has a distinct observational bias, favouring the planets that are easiest to find: massive, Jupiter-like worlds that orbit close to their star. Radial velocity is also biased towards stars rich in heavy elements, since the light from such stars has more spectral lines, making Doppler shifts easier to detect.
Direct imaging
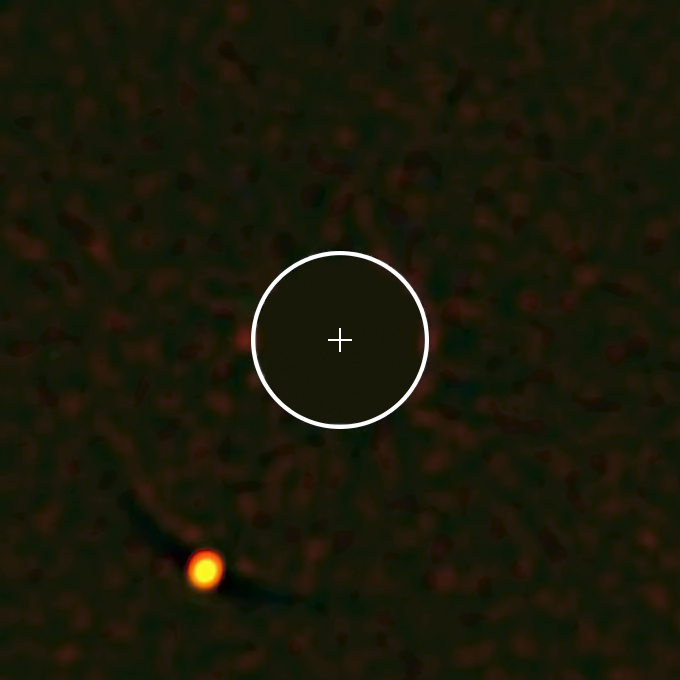
exoplanet HIP 65426b,
produced by ESO’s Very
Large Telescope. The planet’s
star, shown by a cross, has
been masked out. The circle
indicates the orbit of
Neptune on the same scale.
ESO
The ultimate proof of an exoplanet’s existence is a picture of it, but direct imaging requires telescopes with incredibly high resolution. The further away an exoplanet is, and the closer it is to its star, the wider the telescope’s collecting mirror or lens needs to be to resolve the two objects as separate. An 8 m-wide telescope would be needed to separate Jupiter from our Sun when viewed from 600 light years away, while resolving Earth would require a 39 m telescope. A telescope of exactly this size – the European Southern Observatory’s (ESO’s) Extremely Large Telescope (ELT)w2 – is currently under construction in the Atacama Desert of Chile and is due to start searching for exoplanets in 2026. Even greater resolutions can be achieved by combining data from several telescopes arranged over a wider area – a technique known as interferometry.
The bigger challenge to be mastered is the extreme contrast between the brightness of the exoplanet’s reflected light and that of the host star, which is up to ten billion times brighter than an Earth-like exoplanet. One way to overcome this problem is by using masking techniques called coronagraphs to suppress the starlight.
More than 100 exoplanets have now been imaged directly (Chauvin et al., 2017; see figure 3). As with the transit method, direct imaging allows us to study the spectra of light from exoplanets and identify the elements in their atmospheres.
Future developments
The coming years are likely to be very exciting for exoplanet research as new telescopes become operational and detection methods improve further. One promising project in development is SPECULOOS, a set of four 1 m-wide robotic telescopes under construction in the Atacama Desert. SPECULOOS will search for Earth-like exoplanets orbiting near stars with surface temperatures below 2500 K.
In the 2020s, the James Webb Space Telescope (a partnership between NASA, ESA and the Canadian Space Agency) and large-aperture telescopes such as the ELT will provide the resolving power needed to find many more Earth-like planets in the habitable zones around Sun-like stars. Spectral analysis and other image-processing techniques will make it possible not only to identify such worlds but also to perform chemical analysis of their atmospheres – and look for the telltale signs of life as we know it.
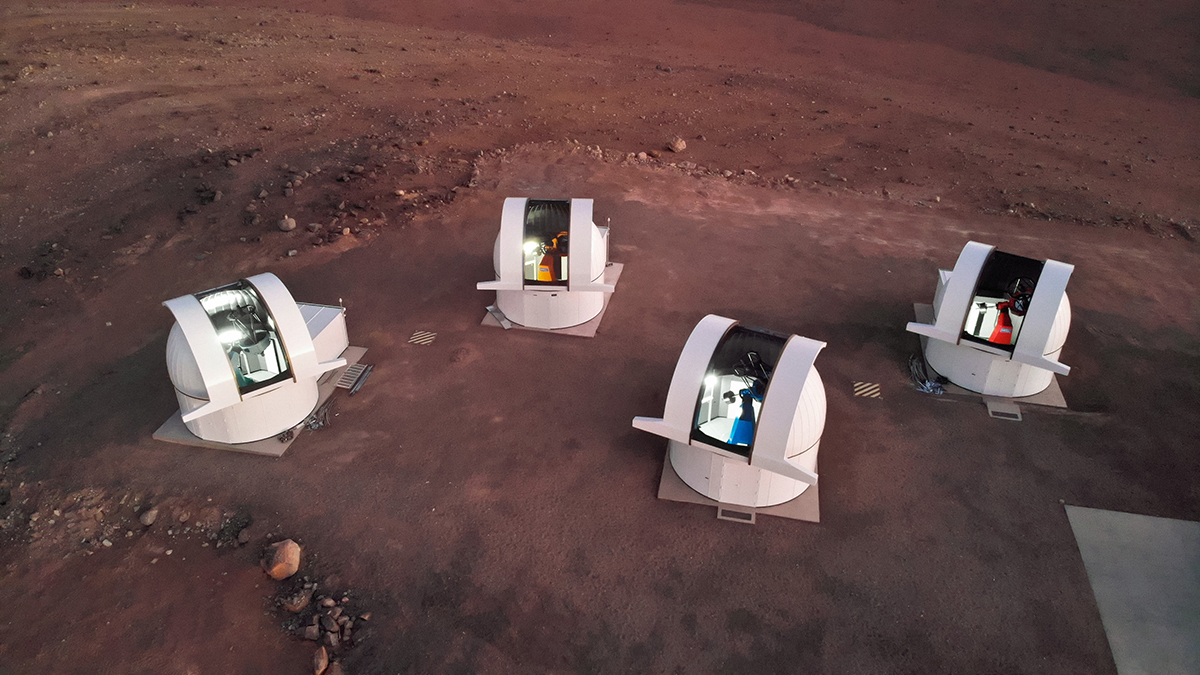
tau-tec GmbH
Exoplanets for schools
The hunt for exoplanets is not just the preserve of professional astronomers with large and expensive equipment. Using the transit method, even a modest 10–20 cm telescope can reveal the eclipse of a star as an exoplanet passes in front of it. Amateur astronomers and schools might be unlikely to discover new exoplanets, but by making follow-up observations of known planets, you could provide invaluable data in our quest to learn more about these fascinating worlds. You can find links to websites describing such activities for schools in the resources section. Happy hunting!
References
- Chauvin G et al. (2017) Discovery of a warm, dusty giant planet around HIP 65426. Astronomy & Astrophysics 605 (L9): 1-9. doi: 10.1051/0004-6361/201731152
- Tsiaras A et al. (2019) Water vapour in the atmosphere of the habitable-zone eight-Earth-mass planet K2-18 b. Nature Astronomy. doi: 10.1038/s41550-019-0878-9
- Wolszczan A, Frail DA (1992) A planetary system around the millisecond pulsar PSR1257 + 12. Nature 335: 145-147. doi: 10.1038/355145a0
Web References
- w1 – Michel Mayor and Didier Queloz were awarded the Nobel Prize in Physics 2019 for their discovery of an exoplanet orbiting a solar-type star. Read about their discovery on the Nobel Prize organisation’s website.
- w2 – ESO is the foremost intergovernmental astronomy organisation in Europe and the world’s most productive ground-based astronomical observatory, with its headquarters in Garching, near Munich in Germany, and its telescopes in Chile.
Resources
- Find out how amateur astronomers can detect exoplanets.
- Learn how amateur astronomers are helping scientists detect more exoplanets. See:
Institutions
Review
The search for exoplanets seems to be a new discipline in astronomy. For the inhabitants of Earth, it is interesting to see if there is something out there similar to our own planet. Perhaps soon we will be able to answer the question: are we alone?
This article outlines the current methods used to discover exoplanets, which employ familiar principles of physics and chemistry. The article could be used as a comprehension exercise, and the following questions and challenges could be included:
- What is an exoplanet?
- Summarise the history of exoplanet discovery.
- Describe one of the methods used to discover exoplanets.
- What is the likely future of exoplanet discovery?
Gerd Vogt, physics and technology teacher, Higher Secondary School for Environment and Economics, Yspertal, Austria