Discovery and controversy: stories from the chemical elements Understand article
How do discoveries happen in science? Despite popular stories of ‘eureka moments’, the reality is usually much more complex.
We are all familiar with stories of scientific advances that climax with a ‘eureka moment’, when the heroic scientist makes the famous discovery. Like the legend of Archimedes – who is said to have shouted ‘eureka!’ after discovering the laws of buoyancy in his bath – these stories typically focus on one brilliant person, at one particular time and place.
The Russian chemist Dmitri Mendeleev’s discovery of the periodic system is often described in this way – with all the elements suddenly falling into a meaningful order according to their atomic weight and chemical properties. In fact, the process of constructing the periodic table took many decades and included plenty of scientific developments that were ultimately dead ends. So what is scientific discovery, and how closely do the legends depict the historical facts?
In this article, we take a closer look at the reality of scientific discovery, with particular reference to the stories of some chemical elements. As well as bringing to light some lesser-known aspects of these discoveries, these accounts illustrate the inherent complexity of any story of discovery within science, both historically and today.
Discovery and nationality
One frequent area of complexity is the attribution of a chemical element’s discovery to a specific country. This idea is depicted in figure 1: a version of the periodic table showing the country of discovery for each element.
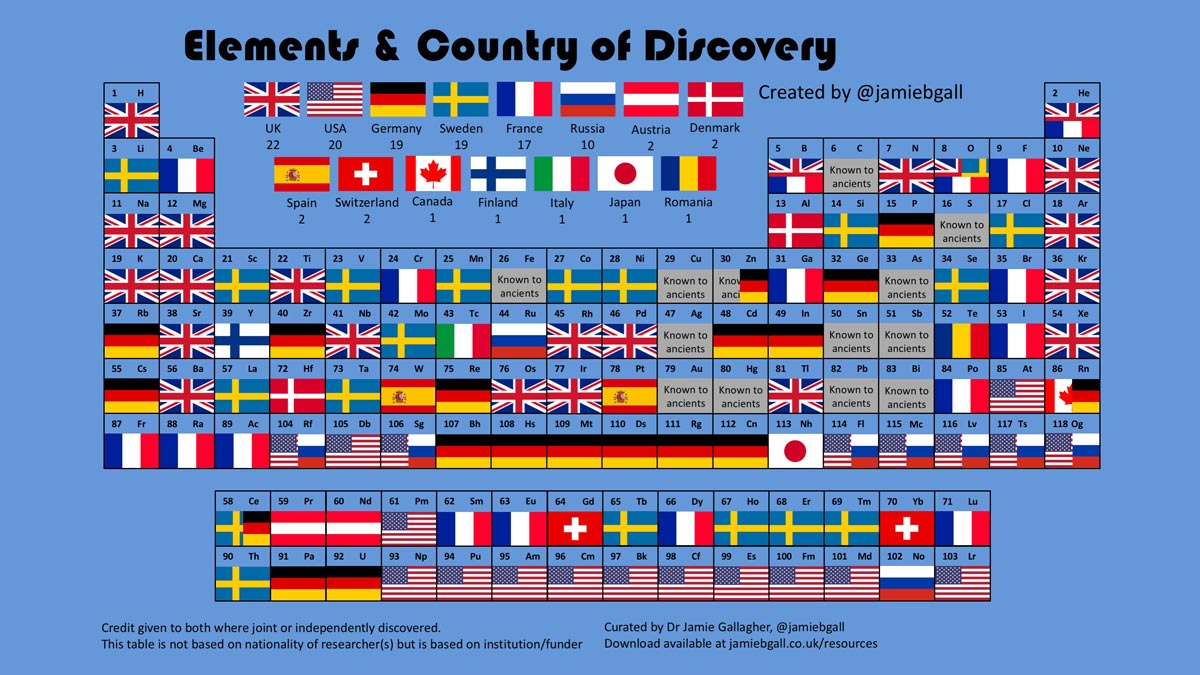
Jamie Gallagher @jamiebgall
Such depictions suggest that there is an exact moment of discovery for each element (perhaps with an underlying sense of national competition). Here, for example, radium (88) and polonium (84) are shown as French discoveries. While it is true that the discoveries took place in France, the principal scientist, Marie Skłodowska Curie, was Polish, and the pitchblende mineral from which the new substances were extracted came from a town now in the Czech Republic, which was then part of the Austria-Hungary empire.
The moving geopolitical map also causes ambiguities in the case of the element tellurium (52). This is shown with a Romanian flag, because Franz-Joseph Müller von Reichenstein, who first suspected the presence of an unknown metal in the ore from which this element was later extracted, and the ore itself, were both from what is now Romania. However, Romania was then part of the Austria-Hungary empire. The attribution of the discovery to von Reichenstein is also controversial, as tellurium was first isolated in 1789 by the German chemist Martin Heinrich Klaproth, who also proposed the name tellurium (Weeks, 1968). But attributing the discovery to Germany would also be problematic, as Germany as a nation did not exist until more than 80 years later.
Oxygen: one discovery – or three?
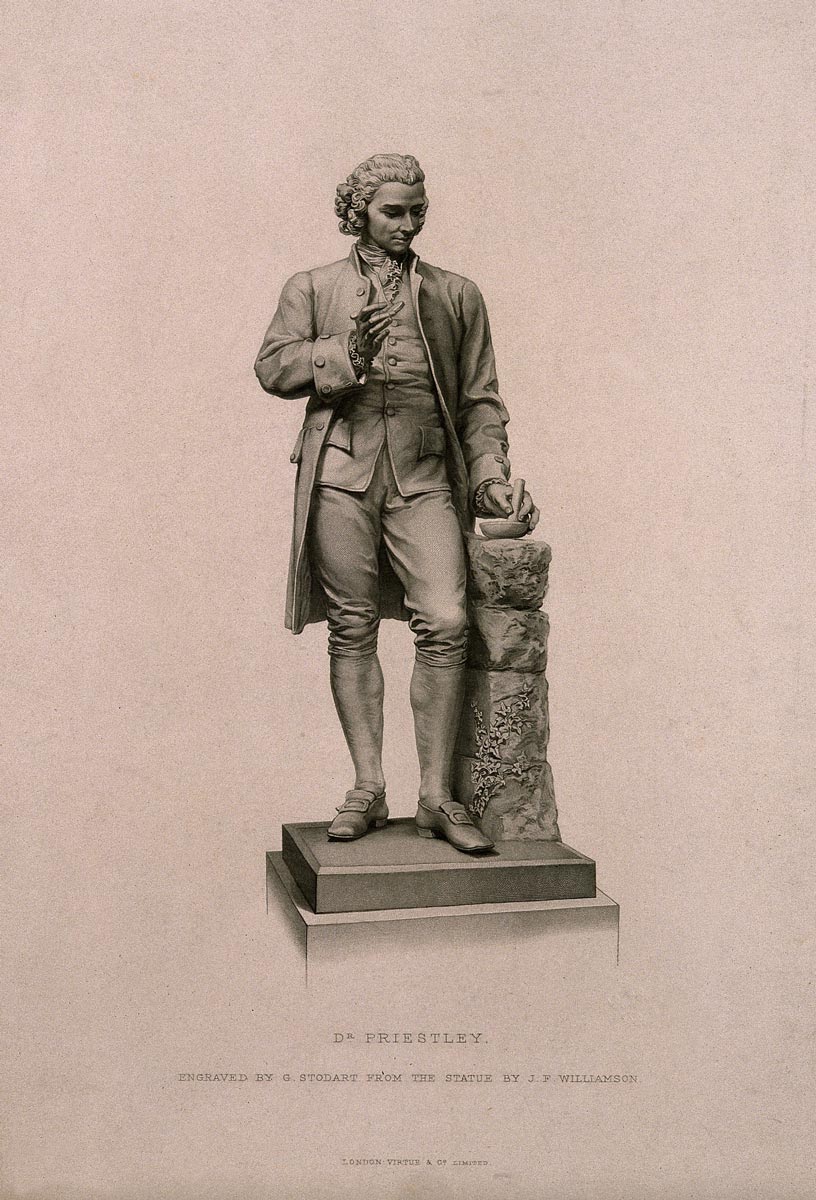
Wellcome Collection, CC BY 4.0
One element for which the controversy of discovery is generally acknowledged is oxygen. The British chemist Joseph Priestley characterised several types of ‘air’, or gas, between 1772 and 1780. When he heated so-called red calx of mercury (HgO), he obtained a type of air that was pleasant to breathe, and which supported combustion much better than normal air – or any other known type of ‘air’. He called the gas ‘dephlogisticated air’, since it was believed that phlogiston was given off from substances when they burned. Because the new kind of air was able to support burning much better than any other air, it was obviously completely devoid of phlogiston.
Antoine-Laurent Lavoisier, the Frenchman who reformed chemistry at the end of the 18th century, was also experimenting with ‘airs’. When they met in 1774, Priestley told Lavoisier about his experiments before they had been published. Lavoisier repeated the calx of mercury experiment and came to the same conclusion: a new gas had been produced. However, he refused to accept the phlogiston theory, naming the gas ‘oxygen’ instead (which means ‘acid producer’ in Greek). Lavoisier viewed oxygen as an element – that is, one of the basic constituents of matter – and launched the oxygen theory of combustion, which is still used today.
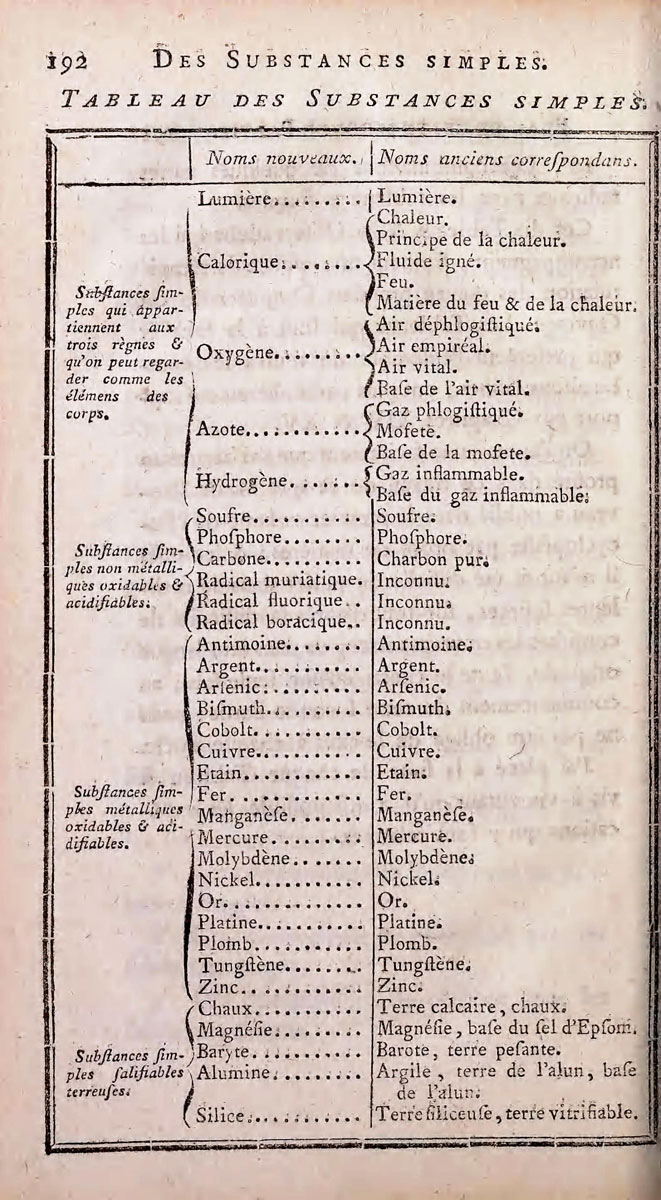
Élémentaire de Chimie,
showing the new and old
names of proposed elements,
or substances simples. Click
on image to enlarge.
Wikimedia Commons/public
domain
A few years before Priestley and Lavoisier carried out their experiments, the Swedish chemist Carl Wilhelm Scheele had discovered the same type of ‘air’, calling it ‘fire air’, since it supported combustion so well. However, he failed to publish his findings until 1777.
So, who should be rightly credited with the discovery of oxygen, and for what achievement? Scheele, who first carried out the experiment to obtain the new gas; Priestley, who first published work about on the new ‘air’; or Lavoisier, who placed the gas in the context of the new chemistry and who finally identified it as an element? In figure 1, all three flags appear in the place for oxygen (8) in the periodic table, demonstrating that this controversy has yet to be resolved.
The controversy surrounding the discovery of oxygen has even been brought to life in a stage play (Djerassi and Hoffmann, 2001), in which the three protagonists (with their life partners), in imaginary reunions of the Nobel committee, tackle the question of who should be credited with the discovery.
Radon: elements and isotopes
The discovery of radioactivity at the turn of the 20th century quickly brought to light a wealth of new radioactive substances. However, it took time to investigate the newly discovered substances – and to work out what radioactivity really was. Mendeleev had built his periodic system on the principle that elements were stable entities, but by 1902 physicists were suggesting that radioactivity transformed one elemental atom into another – a kind of modern alchemy. The initial problems associated with interpreting the empirical facts are illustrated by the story of radon.
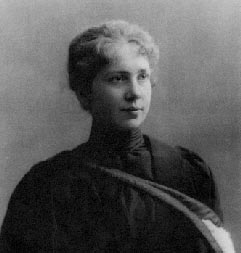
Wikimedia Commons, CC BY-
SA 3.0
In 1899, Ernest Rutherford, working at McGill University in Montreal, Canada, observed that thorium gave off an ‘emanation’ that made the air around it radioactive. The following year, German physicist Friedrich Ernst Dorn showed that radium also generated an emanation. (The Curies had made a similar observation earlier.) Dorn looked for new, unknown spectral lines in the emanation, showing that he suspected it might be a new element. Rutherford then began to examine the nature of the radium emanation systematically (Malley, 2011). Working with his graduate research student, Harriet Brooks, he described the emanation from radium sources as a gas of heavy molecular weight, which could not be a vapour of radium, with the implied conclusion that this was an unknown radioactive gas. The proof that this was indeed a new noble gas element came in 1910, when William Ramsay and Robert Whytlaw-Gray produced a unique spectrum similar to the spectra of the inert gases (Marshall and Marshall, 2003).
Today, we know that the thorium emanation was the radon isotope Rn-220, and the radium emanation the radon isotope Rn-222. In the decades to follow, other isotopes of radon were reported, generating a wide variety of names, including: acton, actineon, actineon, exradio, exradium, exthorio, exthorium, exactinio, niton, radeon, radon, thoreon and thoron. The name ‘radon’ was adopted officially only much later, in 1931, and the term ‘emanation’ could still be found in scientific contributions as late as the early 1960s.
So, who did discover radon and what stage in the long and complex discovery process should count as discovery? Historians and chemists have struggled to set the record straight, suggesting Curie, Dorn, Rutherford or Ramsay and Whytlaw-Gray as discoverers of radon. Harriet Brooks, the graduate student whom Rutherford acknowledged for realising that the emanation was a radioactive gas, is, however, almost never credited (Rayner-Canham and Rayner-Canham, 2004).
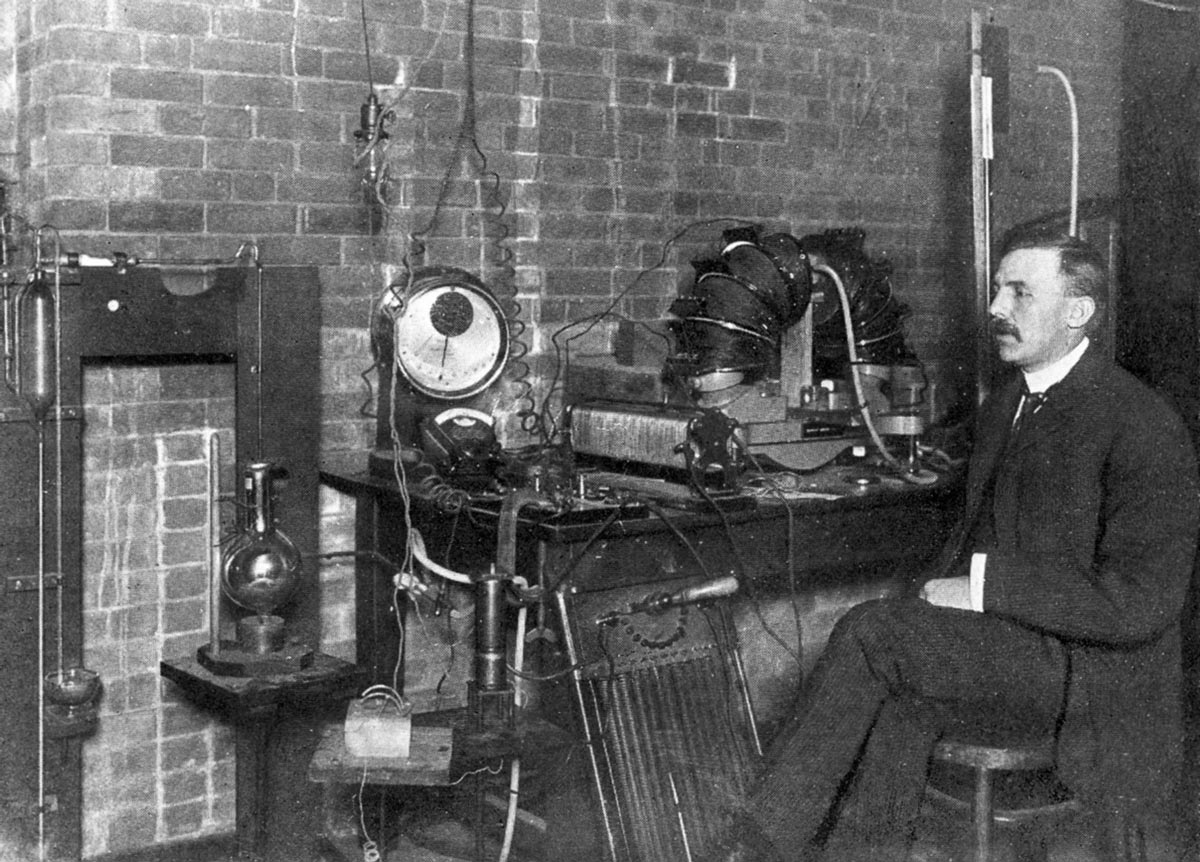
Wikimedia Commons, CC BY 4.0
Astatine and artificial synthesis
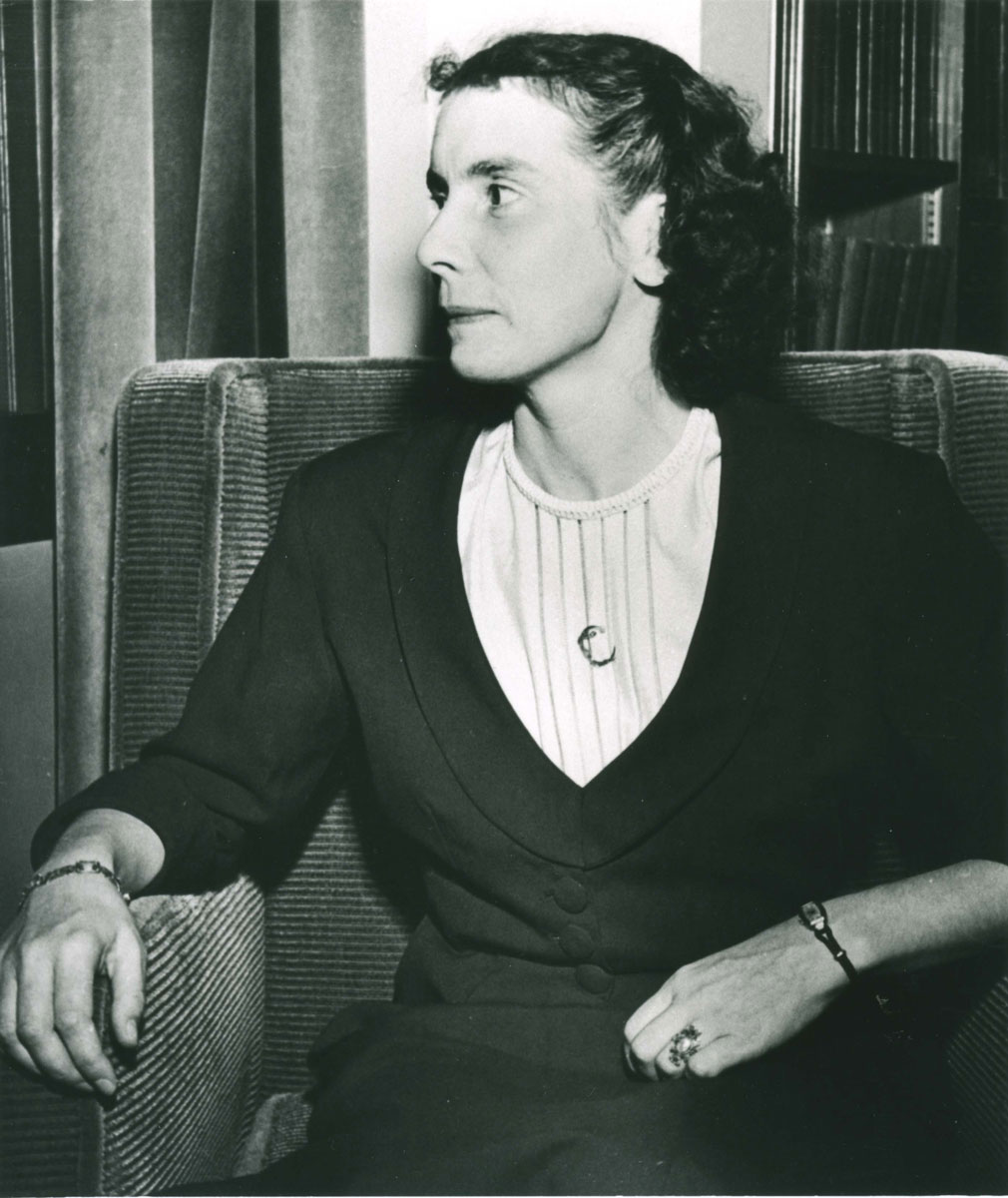
Musée Curie (coll. ACJC)/1857
In many cases, progress in identifying new elements has depended on technical developments. In 1932, the Frenchwoman Yvette Cauchois developed a type of spectrometer that made it possible to study the spectra of elements that produced weak spectral lines. Cauchois and her Romanian colleague Horia Hulubei found new spectral lines in the radioactive decay of radon that they argued were typical of an element with atomic number 85, which at that time had not been detected.
A few years later in Vienna, an all-female Austrian team, Berta Karlik and Traude Bernert, detected two naturally occurring isotopes of element 85 and published their findings in 1942 and 1943 (Lykknes and Van Tiggelen, 2019). What the French-Romanian and Austrian researchers did not know, however, was that atoms of element 85 had already been synthesised at Berkeley, California, in 1940. The scientists behind this research (Dale Corson, Kenneth MacKenzie and Emilio Segre) used a cyclotron to synthesise the element, bombarding bismuth-209 with alpha particles.
In 1947, in an article in Nature devoted to missing chemical elements, the prominent chemist Friedrich Paneth suggested that elemental discoveries should, as a general rule, be credited to the first team who succeeded both in synthesising and characterising one or more isotope(s) of the new element. According to Paneth, in the case of element 85 this was undisputedly the American team, who were therefore entitled to name the new element; they chose the name astatine, from the Greek astatos, which means ‘unstable’.
Competition and consensus
Paneth also provided an authoritative review of the discoveries of another seven elements (43, 61, 87, 93, 94, 95 and 96), setting out guiding principles for who has the right to name a new element: this should go to the first scientists to provide definite proof of the existence of one of the element’s isotopes, with no discrimination between naturally occurring and artificially produced isotopes. These principles were adopted by the scientific community in 1947. Producing, observing and identifying elements, and providing proof of their existence, were now all part of the same enterprise.
However, like today, there were only a few places where the technology to make new elements was available: the Lawrence Livermore National Laboratory in the USA, the GSI Helmholtz Centre for Heavy Ion Research in Germany, the Joint Institute for Nuclear Research in the USSR (later Russia), and RIKEN in Japan. But while there is now more agreement on what constitutes a discovery, the controversies are no less fierce, as these laboratories rely on each other for confirmation of their findings, while at the same time competing.
Scientific discovery in context
Scientists from all disciplines know, from their daily practice, that discovery is a process. In relation to recent cases in astronomy of finding a new exoplanet, for example, one planetary discoverer has commented, “It’s not like there’s a single moment of discovery”w1. In this case, as so often, the ‘discovery’ was only apparent within the context of the overall research, requiring a sizeable team and decades of experience and development. Science is not an enterprise propelled forwards by some lonesome genius – nor does it follow a linear narrative. In the history of science, detail and complexity are always part of the story.
References
- Djerassi C, Hoffmann R (2001) Oxygen. Weinheim, Germany: Wiley-VCH. ISBN: 9783527304134
- Lykknes A, Van Tiggelen B (eds) (2019) Women in their Element: Selected Women’s Contributions to the Periodic System. Singapore: World Scientific. doi: 10.1142/11442
- Marshall JL, Marshall VR (2003) Ernest Rutherford, the ‘true discoverer’ of radon. Bulletin for the History of Chemistry 28(2): 76-83
- Malley M (2011) Radioactivity: A History of a Mysterious Science. Oxford, UK: Oxford University Press
- Rayner-Canham MF, Rayner-Canham GW (2004) Rutherford, the ‘true discoverer of radon’. Bulletin for the History of Chemistry 29(2): 89-90
- Weeks ME (1968) Discovery of the Elements. 7th edition. Easton, PA, USA: Journal of Chemical Education Resources
Web References
- w1 – Quote from NASA intern Wolf Cukier (age 17), after discovering a previously unknown planet 1300 light years from Earth.
Resources
- Read about other pioneering scientists whose work contributed to the development of the periodic table.
- Lykknes A, Van Tiggelen B (2019) In their element: women of the periodic table. Science in School 47: 8-13
- Explore another version of the periodic table showing the country of discovery for each element, on the Open Culture website.
Review
Within science teaching, attribution of a discovery to an individual is not unusual, but so often the bigger picture, the ‘story’ behind the discovery, tends to be overlooked. I am always keen to learn the stories behind discoveries in chemistry, as these can be used to such good effect, making the abstract real in the classroom. The human experiences behind scientific discoveries not only make them less dry, but also help young students to remember and recall more information.
This article provides a series of very short stories about particular elements and their discovery. It has international appeal and would make excellent background reading for students aged 16–18 or as a discussion prompt for ages 14–16 and 16–18. As well as being used when discussing how science works or the discovery of new elements, the article could be useful in a discussion of competition in science. It could also be linked with geography and history when discussing the attribution of a discovery to a specific country amidst a shifting geopolitical map.
Caryn Harward, head of chemistry, St Mary’s School, Calne, UK.