Wind and rain: meteorology in the classroom Teach article
Why does it rain? Can we predict it? Give physics students a mass of weather data and some information technology, and they can try working this out for themselves.
Thermodynamics is taught in high-school physics – but how many students make the connection with our everyday weather, even though both are governed by the same variables (temperature, pressure and so on)? We decided to try to make this connection clear to students by asking them to explore for themselves how such variables affect each other in the environment, using real scientific data.
By the end of the activity, the students were impressed by the logical way in which these natural phenomena are related – and got a thrill from working with a top scientific institution, the National Observatory of Athens, Greece.
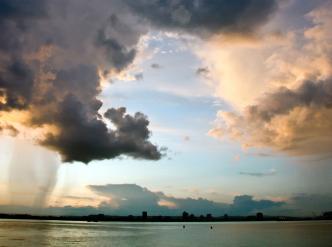
Aims
Our aim was to extend the high-school study of thermodynamics to Earth’s most important gaseous mixture: the atmosphere. So we devised an activity where students used real data from a weather station to investigate how temperature and pressure interact in Earth’s atmosphere, and how these variables are correlated with other important physical quantities (relative humidity, dew point and wind direction).
In this article, we provide details of our activity and show our own results as an example. We hope you will be inspired to repeat this activity using local data from your own region.
The activity itself takes about 6 hours, although you may need to allow up to 4 more hours to ensure that students have all the background knowledge they need before they start.
Preparation
Before starting the activity, students should have classroom knowledge and skills in the following areas:
- Gas laws and the variables involved
- Other thermodynamic variables (relative humidity, dew point) and theories of how they are related to temperature and pressure
- Basic meteorology (allow 2 hours to teach this, if needed). This should include: high and low pressure systems and the weather conditions they cause; air masses and fronts; global winds and jet streams; and how to read a weather map. Students should also be able to draw logical conclusions about everyday weather phenomena, such as ‘When the wind comes from the sea, the relative humidity increases’. (The resources section at the end of this article contains links to useful videos for teaching these topics.)
- Ability to plot graphs using computer programs. Microsoft Excel is ideal for this, as it is easy to extract data from tables and represent them graphically. Allow 2 hours to teach this if needed (this can be done in a science or information technology lesson).
Finding a suitable source of data. There are two options here: either contact a weather organisation that may be willing to provide data from a previous or current year (our source was the National Observatory of Athens) or install your own school meteorological station. Either way, you will need data for all the variables you wish to study. We suggest the following variables:
- Atmospheric pressure
- Air temperature
- Relative humidity
- Dew point
- Wind direction
The amount of data you need will depend on what you wish to study. For example, if you wish to study changes in air temperature in a one-month period, you will need 1-2 readings per day for 30 days. Alternatively, if you wish to study changes in air temperature from day to night, you will need readings taken every 30 minutes for a few days.
We used data from winter, because in the Mediterranean region, winter is the most interesting season meteorologically, as it shows the greatest changes in the values of the physical variables we studied.
Procedure
- Divide the students into groups so that each group can work on a different set of data. Initially, we suggest that different groups work on the data for different variables in the same period, as shown in the graphs below. As an extension, data from different periods can also be used.
- Provide the students with their group’s data, and instruct each group to create one or more graphs from the data. They should plot the variables either against each other or against time (t) for the whole period covered by the data.
- Ask students to decide what correlations they can find between the variables and to express these as clearly as possible. Using their knowledge of meteorology, can they suggest what might cause these correlations?
- As a final task, students can try to predict the weather in their own or another area, using current data and the principles they have learned about in this activity.
Example results
To explain how to use the data, we present some examples of the graphs produced in our classroom for December 2014, together with explanations of what they revealed.
The National Observatory of Athens provided us with data from a weather station between the Ionian Sea (part of the Mediterranean) on the south-west side of the station, and a mountain located in an east-south-easterly direction from the station (figure 1). This was ideal, as it meant that wind blowing from different directions had a direct influence on the weather.
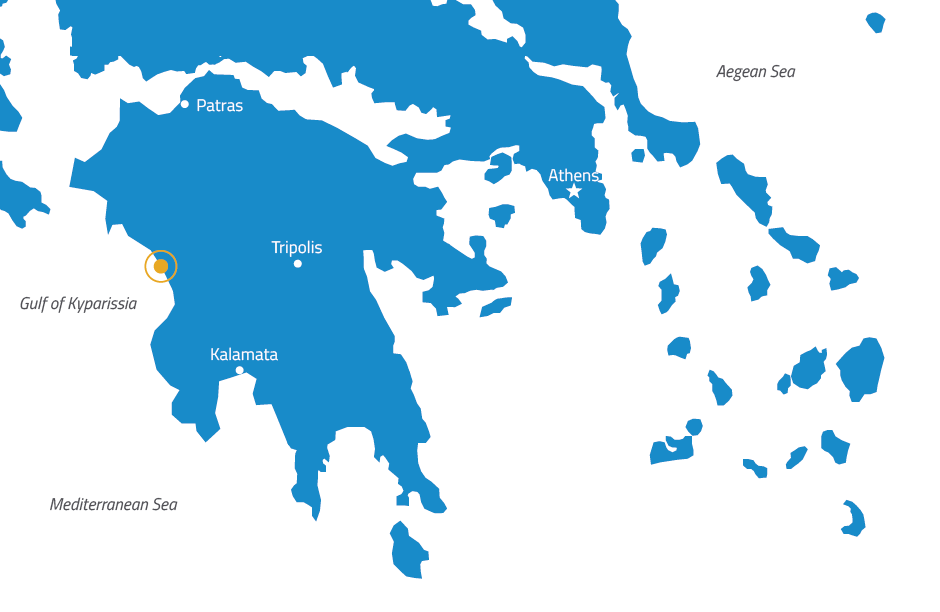
station indicated by a an orange circle.
To show the wind direction on the graphs, the 16 different compass points (S, SSW, SW, WSW, etc.) were each allocated a different colour, as shown in figure 2 and table 1. (Note: The wind direction is where the wind blows from, not towards.)
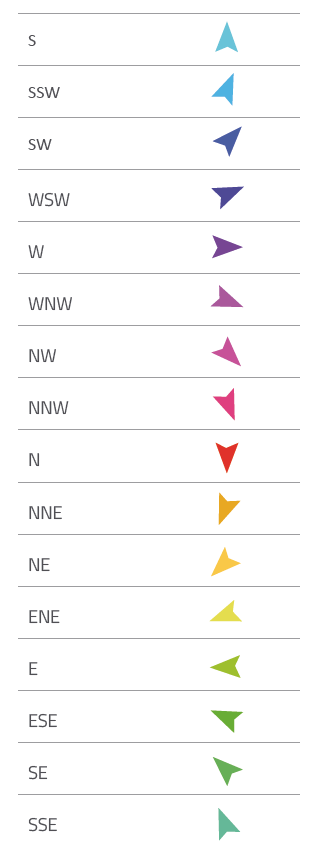
their corresponding colours
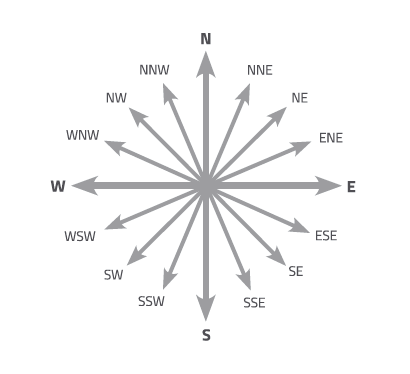
Image courtesy of Nicola Graf
Wind direction and air temperature
From figure 3, we can see the following correlations:
- When southerly and east-south-easterly winds prevail, there is an increase in air temperature. This is seen at the region of the graph labelled A. Such winds are known as descending winds because they come largely from the direction of the mountain and are naturally warm and dry. This is because air warms as it descends the mountain, and it is also dry, having lost much of its moisture on the windward side of the mountain.
- The temperature fluctuates between night and day. It is cooler at night and warmer during the day. This can be seen at points B and C on the graph.
- Land and sea breezes produce mild weather. When there are humid winds during the day and dry winds during the night, it means daytime sea breezes (here from the west or south-west, as at point D) and evening land breezes (here from the east), which produces mild weather.
Another phenomenon, which is not apparent from the graphs but which we often notice, is that when there is a wind from the north, air temperature falls. The northerly wind causes a drop in air temperature because it comes from regions closer to the North Pole, which are colder than ours.
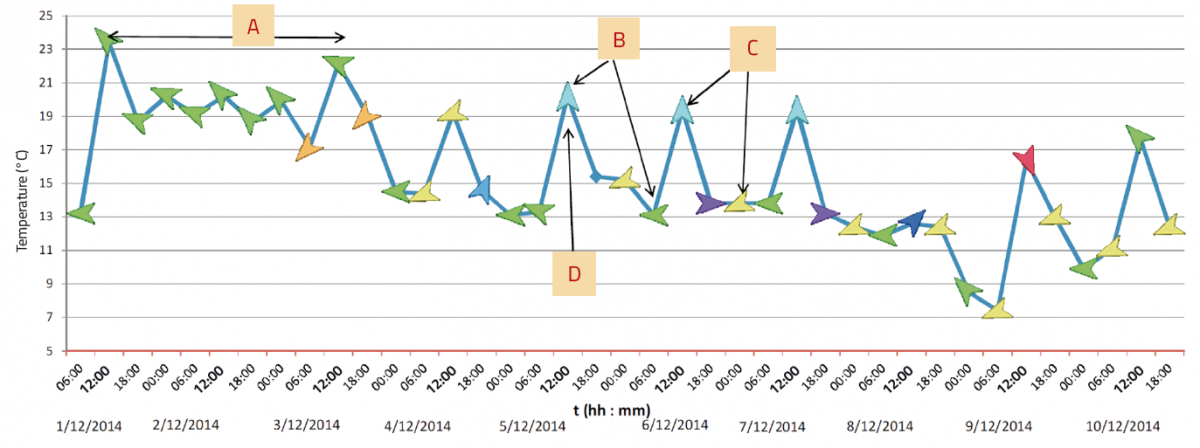
Image courtesy of Maria Birba and Theodoros Kondilis
Wind direction and relative humidity
Wind carries a different amount of humidity depending on where it has come from (for example, over land or sea). From figure 4, we can see the following correlations between wind direction and relative humidity for our location:
- When easterly (descending) winds prevail, relative humidity decreases. This (seen at A and B on the graph) is because the winds from the mountains are warm and dry.
- When the winds are southerly or south-westerly, relative humidity increases significantly. This (as at C) is because these winds come from the sea.
- Slight fluctuations in humidity are linked to winds shifting direction. These winds (seen at interval D) are also likely to be weak.
- Relative humidity varies with time of day. Relative humidity is normally high at night (so we see condensation) due to cooler temperatures (see point E on the graph), and low during the warmer daytime hours (revealed by evaporation; see point F). When clouds are absent during the night, more heat is lost from Earth’s surface through radiation, producing cooler temperatures and increased humidity.
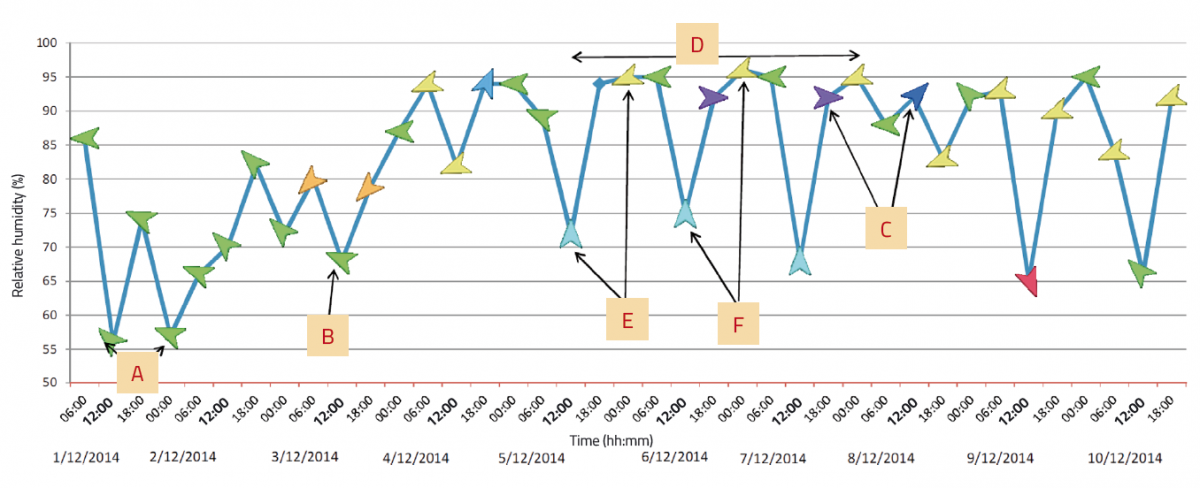
Image courtesy of Maria Birba and Theodoros Kondilis
Relative humidity, air temperature, dew point and rainfall
From figure 5, we can see the following correlations:
- Changes in air temperature are linked to changes in relative humidity. This is because for the same amount of moisture in the air, a higher air temperature means a lower relative humidity. So when temperature rises, relative humidity falls.
- The dew point varies less than the temperature. The dew point reflects the concentration of water vapour in the atmosphere. This was less variable than temperature in the location and period that we studied.
- When the air temperature becomes the same as the dew point, rain is likely to fall. This is because, at the dew point, relative humidity reaches 100%, leading to condensation.
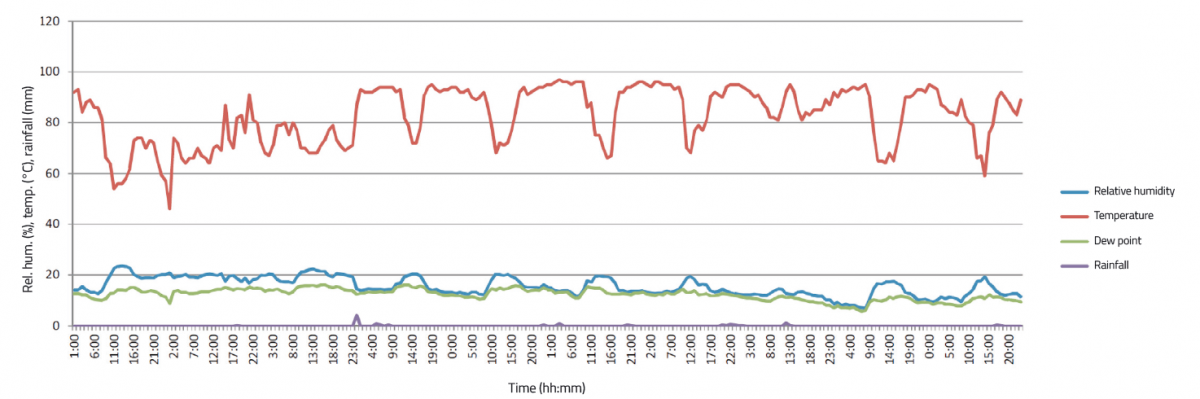
Image courtesy of Maria Birba and Theodoros Kondilis
Atmospheric pressure and air temperature
From figure 6, we can see the following correlations between temperature and atmospheric pressure in high- and low-pressure conditions:
- Low pressures alter the temperature. When a low barometric pressure approaches a region, intense winds are drawn in towards the centre of this system because the pressure is lower inside the system than outside it. If the prevailing winds are from warmer regions, then the temperature increases. This phenomenon is called thermal intrusion. It occurs frequently at our weather station, where low-pressure systems from a south-south-westerly direction (the Mediterranean) arrive and increase the temperature (as at A, B and C on the graph). If the prevailing winds are from colder regions, this is called a cold intrusion and the temperature normally decreases – as seen at G, where a very low-pressure system caused a rapid decrease in temperature on the last two days of the month.
- High pressures bring fine weather. The opposite phenomena are observed when a high barometric pressure approaches a region: winds are very weak or completely absent and the sky is cloudless, meaning fine weather. This is seen here at intervals D and E.
- High-pressure systems cause temperature inversion. The absence of clouds increases heat loss by radiation from Earth’s surface, thus cooling both the surface and the lower levels of the atmosphere (seen at the points indicated by arrows at F). This leads to cooling of the lowest levels of the atmosphere relative to those just above, hence the idea of ‘inversion’.
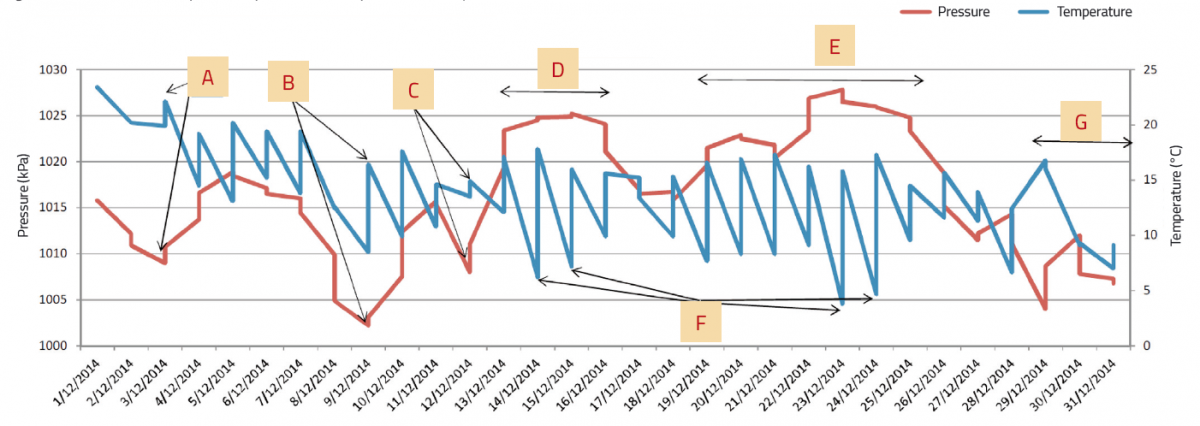
Image courtesy of Maria Birba and Theodoros Kondilis
Atmospheric pressure and rainfall
From figure 7, we can see the following correlations between atmospheric pressure and rainfall:
- When the atmospheric pressure falls, rainfall occurs. When a low barometric pressure approaches, it is accompanied by intense winds. These winds move anti-clockwise, converging at the centre of the system and then rising, as they have nowhere else to go. The air masses cool as they move upwards, so condensation takes place, clouds are formed and precipitation occurs. We can see this on the graph at the points indicated by arrows at A.
- When the atmospheric pressure is high, rainfall is unlikely. When a high-pressure system approaches, winds are either absent or weak. The air masses converge high up and descend, moving to the centre of the system. They become warmer as they fall, so water droplets evaporate rather than condense. The result is that there are no clouds, so no precipitation and the weather is fine. This can be seen at B on the graph.
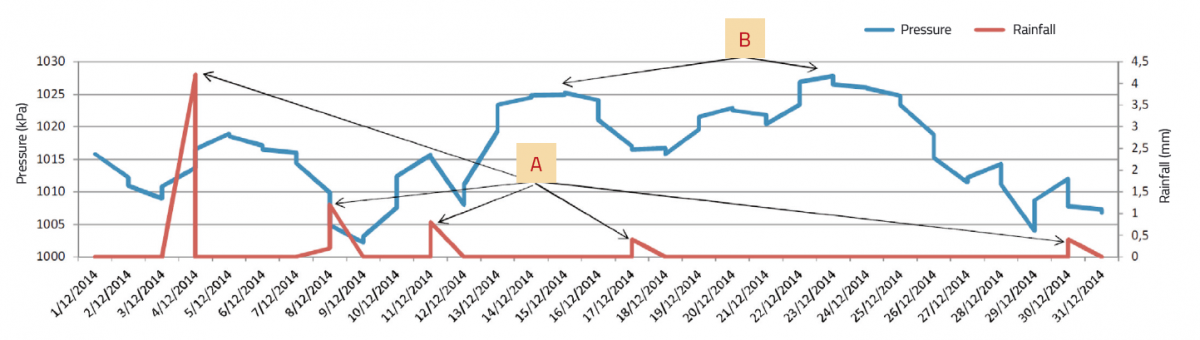
Image courtesy of Maria Birba and Theodoros Kondilis
End note
During their investigations, the students worked with great zeal as they became thoroughly involved in doing something rather unusual for a science lesson. The teachers encouraged students to make connections between their everyday experiences and their existing scientific knowledge – so by increasing their understanding of common weather phenomena, the students also extended and deepened their knowledge of thermodynamics.
Impressively, the students were able to use their new knowledge to make some valid short-term weather predictions. As a simple example, from noticing an increase in atmospheric pressure and temperature, they predicted 1–2 days of fine weather without rain – quite correctly.
Acknowledgements
The authors would like to thank Nickolaos Zounis and Susan Watt for their help in preparing this article and in creating the graphs.
Resources
- For explanatory videos on the following meteorological topics, see the web links:
- For videos about using information technology to create graphs, see these web links:
- Ahrens CD (2013) Meteorology Today, an Introduction to Weather, Climate, and the Environment 10th edition. Belmont, Canada: Cengage Learning. ISBN: 978-1285114163
- Glickman S (2000) Glossary of Meteorology 2nd edition. Boston, USA: American Meteorological Society. ISBN: 978-1878220349
- Schaefer VJ (1998) Peterson First Guide to Clouds and Weather 2nd edition. Boston, USA: Houghton Mifflin Harcourt. ISBN: 978-0395906637
- Wallace JM, Hobbs PV (2006) Atmospheric Science: An Introductory Survey 2nd edition. Burlington, MA, USA: Elsevier Academic. ISBN: 978-0127329512
Review
This article describes an enjoyable way to use various detailed weather data sets to consider thermodynamics in everyday actions and to predict the weather if the data sets are local enough to the school using them.
Usually this topic is treated at a very basic level, without any particular attempt at extrapolation or prediction, which makes this activity novel in its depth, detail and approach.
Eric Deeson, UK