Sloppy fishing: why meiosis goes wrong Understand article
Why does meiosis so often go wrong? And what are the consequences?
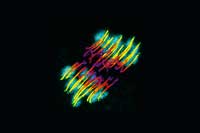
coloured lines chart the
movement (purple to yellow)
of kinetochores (green dots)
as microtubules hook onto
them to separate the
chromosomes (cyan).
Image courtesy of Tomoya
Kitajima, EMBL
At a village fair, past the bumper cars and candy-floss, a child’s forehead creases in concentration. He’s doing his best to snag a wooden fish out of a plastic pond, using the magnet on the end of his fishing rod. Freeze the scene, rewind all the way through the child’s development, past the point when the sperm fertilised the egg, to when the egg cell itself was formed, and you’ll find a similar fishing game in action. The difference, as scientists at the European Molecular Biology Laboratory (EMBL; see box) have found, is that magnet-wielding children are probably more successful fishermen than the egg cell’s machinery (Kitajima et al., 2011).
As an egg cell, or oocyte, matures inside a woman’s ovary, it undergoes a type of cell division called meiosis, in which the pairs of chromosomes inside it are lined up and fished apart, and half of them are expelled. The chromosomes are brought together from all over the cell (Mori et al., 2011) and fished apart by protein rods called microtubules. Like the child’s rod pulling a toy fish by its magnet, a microtubule catches a chromosome by its kinetochore – a cluster of protein and genetic material at the centre of the chromosome’s X shape.
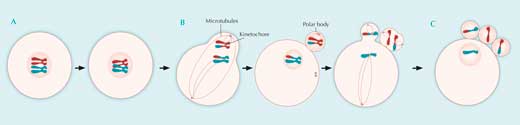
A: During the first five months of development of a female human embryo, all its potential future egg cells are formed. In each of these cells, after DNA duplication, homologous chromosomes exchange genetic material during crossing over. Meiosis is then halted until ovulation, and most of the potential egg cells die off again.
B: Between puberty and menopause, during each monthly cycle, a few potential egg cells progress further during the stages of meiosis, but only one at a time eventually completes the process. Homologous chromosomes line up at the primary egg cell’s equator and are then fished apart by microtubules. The primary egg cell divides into a secondary egg cell and a polar body.
Now paired chromatids line up at the equators of both the polar body and the egg cell, and at the time of ovulation, microtubules attach to them. Meiosis is arrested here until fertilisation.
C: If fertilisation happens, the paired chromatids are pulled apart, moving to opposite poles of the cells. The polar body divides in two, the secondary egg cell divides into a third polar body and a mature egg cell, and meiosis is complete. Each of the four resulting daughter cells has a different genetic makeup.
The genetic material of the polar bodies is discarded, while that of the mature egg cell is joined by the genetic material of the fertilising sperm, to start the development of a new embryo. Click on image to enlarge.
Image courtesy of Nicola Graf
By examining mouse egg cells under the microscope, EMBL scientist Tomoya (Tomo) Kitajima was the first to track the movements of all of an egg cell’s kinetochores during the whole of cell division – all 10 hours of it. “We were able, for the first time, to keep track of all the kinetochores throughout cell division – so there’s not a single time point where it’s ambiguous where that part of the chromosome is – and that’s really a breakthrough in the field, achieving this in these very large and light-sensitive cells,” says Jan Ellenberg, who heads the research group.
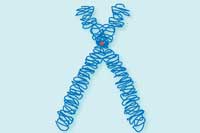
The centromere (red dot) is
the site of assembly of the
kinetochore.
Image courtesy of Tryphon;
image source: Wikimedia
Commons
Tomo used software that had been previously developed in Jan’s lab, which allowed him to programme a laser scanning microscope to find the chromosomes in the egg cell’s vast inner space, and then film them during cell division. “The oocyte is a big cell, but the chromosomes sit in only a small part of that cell, and that’s what we were interested in. So basically we just made our microscopes smart enough that they can recognise where the chromosomes are and then zoom in, in space and time, just on that region,” Jan explains.
By focusing the microscope only on the part of the cell where the chromosomes are, Tomo was able to obtain high-resolution images at short intervals of only one and a half minutes, which gave him a very clear picture of the process. And, because the microscope was only firing light at that small region of the oocyte, it did less damage to the cell, which enabled the scientists to keep up the imaging for the 10 hours of cell division (see box for more on smart microscopy).
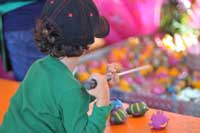
Image courtesy of QUOI Media;
image source: Flickr
Back at the fishing pond, tempers can flare and voices rise in shrill accusation: “That’s cheating! You can’t push the fish with your rod!” Thanks to Jan and Tomo’s work, the accused child could argue, in his defence, that his cells were already ‘cheating’ like this before he was even born. When the EMBL scientists analysed the videos, they found that, before microtubules attach to kinetochores, they nudge chromosomes into a favourable position, like a child repositioning a fish with the end of his rod. The microtubules nudge the chromosome arms, arranging the chromosomes in a ring from which they can then fish them out more easily.
“But even with this pre-positioning, it still doesn’t work very well,” says Jan. “We saw that 90 % of kinetochore connections were initially wrongly established, and the microtubules had to release the chromosome and try again – on average, this had to be done three times per chromosome.”
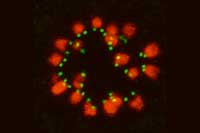
before they start attaching
themselves to kinetochores
(green), microtubules nudge
chromosomes (red) into a
ring around the centre
of the spindle.
Image courtesy of Tomoya
Kitajima, EMBL
Scientists in the USA have now shown that the same ‘cheating’ also happens in the other type of cell division, which our cells undergo when we grow or when tissues such as skin regenerate (Magidson et al., 2011). In this second type of cell division, called mitosis, a cell divides into two daughter cells, each with the same amount of genetic material as the ‘mother cell’, instead of half the genetic material as in meiosis. But Jan and Tomo’s findings highlight that the fishing of chromosomes involves much more error in the egg cell’s meiosis than in mitosis. The greater degree of error in meiosis could, the scientists believe, be due to a fundamental difference in how microtubules fish chromosomes apart in the two types of cell division.
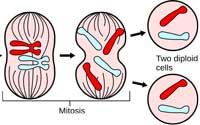
replicated its DNA, the
chromosomes line up at the
cell’s equator. Microtubules
attach to the kinetochores of
sister chromatids and fish
them apart. After mitosis, the
cell divides. The two
resulting daughter cells are
genetically identical to the
parent cell. Click on image
to enlarge.
Image courtesy of Mysid;
image source: Wikimedia
Commons
During mitosis, the microtubule rods start forming at two opposite points in the cell and come together in a lemon-shaped structure – the spindle – that then pulls each chromatid in a pair to one side, or pole. But in meiosis, as Jan’s group discovered a few years ago (Schuh & Ellenberg, 2007), the spindle’s microtubules converge from as many as 80 different points at first, and only later arrange themselves into a two-poled structure. “So when microtubules are first attaching to chromosomes, it’s hard to know if they’re going to end up pulling them in opposite directions or not,” Jan explains. This, along with the fact that the egg cell is a much larger expanse across which microtubules have to find and drag chromosomes – a human egg cell is more than four times larger than a skin cell – could explain why chromosome fishing is so much more error-prone in egg-cell division.
These findings also provide scientists with a more concrete place to look when studying female infertility and conditions like Down syndrome, which largely stem from egg cells with an abnormal number of chromosomes. By showing that such errors most likely occur because microtubules fail to make the right connections to separate chromosomes properly, Tomo and Jan have provided a focus for future studies. In fact, Tomo is now going on to study why this trial-and-error process is even more error-prone in older egg cells. If he and others can pinpoint where the error-correction mechanisms fail in older cells, it could one day be a starting point for medical procedures to help microtubules improve their fishing technique. Perhaps the secret to countering age-related infertility is to make microtubules as successful at fishing as children are with their toy magnets.
Making microscopes smarter
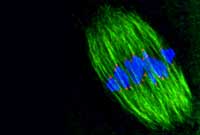
in preparation for separation.
Kinetochores (red) attach
chromosomes to the cell’s
microtubules (green).
Image courtesy of Tomoya
Kitajima, EMBL
The software that Tomo used to find and film chromosomes throughout cell division was a prelude of things to come. Since then, in collaboration with another team at EMBL led by Rainer Pepperkok, Jan’s group has developed a more complex programme, capable of even greater feats of automation. Called Micropilot, the new software analyses low-resolution images taken by a microscope and finds not just chromosomes but whatever structure the scientist has taught it to look for.
Once Micropilot has identified the cell or structure that the scientists are interested in, it automatically instructs the microscope to start an experiment. This can be as simple as recording high-resolution time-lapse videos or as complex as using lasers to interfere with fluorescently tagged proteins and recording the results. The software is a boon to systems biology studies, as it generates more data at a faster pace. Thanks to its high throughput, Micropilot can easily and quickly generate enough data to obtain statistically reliable results, allowing scientists to probe the role of hundreds of different proteins in a particular biological process.
More about EMBL
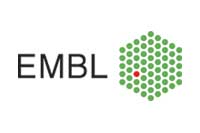
The European Molecular Biology Laboratory (EMBL)w1 is one of the world’s top research institutions, dedicated to basic research in the life sciences. EMBL is international, innovative and interdisciplinary. Its employees from 60 nations have backgrounds including biology, physics, chemistry and computer science, and collaborate on research that covers the full spectrum of molecular biology.
EMBL is a member of EIROforumw2, the publisher of Science in School.
References
- Kitajima TS, Ohsugi M, Ellenberg J (2011) Complete kinetochore tracking reveals error-prone homologous chromosome biorientation in mammalian oocytes. Cell 146(4): 568-81. doi: 10.1016/j.cell.2011.07.031
- Magidson V et al. (2011) The spatial arrangement of chromosomes during prometaphase facilitates spindle assembly. Cell 146(4): 555-67. doi: 10.1016/j.cell.2011.07.012
- Mori M et al. (2011) Intracellular transport by an anchored homogeneously contracting F-actin meshwork. Current Biology 21: 606-61. doi: 10.1016/j.cub.2011.03.002
- A freely available and simply written explanation of this research is available in the EMBL annual report:
- EMBL (2012) Neat nets. In EMBL Annual Report 2011/2012 pp 86-88. Heidelberg, Germany: European Molecular Biology Laboratory.
- Schuh M, Ellenberg J (2007) Self-organization of MTOCs replaces centrosome function during acentrosomal spindle assembly in live mouse oocytes. Cell 130(3): 484-98. doi: 10.1016/j.cell.2007.06.025
- A freely available and simply written explanation of this research is available in the EMBL annual report:
- EMBL (2008) Push me, pull you. In EMBL Annual Report 2007/2008 pp 46-50. Heidelberg, Germany: European Molecular Biology Laboratory.
Web References
- w1 – For more information about EMBL, see the EMBL website.
- w2 – EIROforum is a collaboration between eight of Europe’s largest inter-governmental scientific research organisations, which combine their resources, facilities and expertise to support European science in reaching its full potential. As part of its education and outreach activities, EIROforum publishes Science in School.
Resources
- Watch a video of microtubules nudging the chromosomes into position.
Institutions
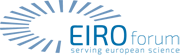
Review
This article is about new scientific inputs concerning the understanding of cell division mechanisms, namely the attachment of microtubules to chromosomes during mitosis and meiosis.
The level of detail in this article makes it particularly useful for upper-secondary biology classes (ages 15+), for topics such as cytology (mitosis and meiosis), genetics (the causes and implications of chromosomal abnormalities) and reproduction (gametogenesis and infertility).
The article can also be used to initiate wider discussions about both the benefits of modelling biological phenomena (models can help us to understand processes) and the risks. For example, in the majority of text-books describing mitosis and meiosis, chromosomes are represented as large structures. This can lead students to believe that chromosomes are easily observable in any type of cells. As is clear from the article, however, this is not the case.
Finally, the article illustrates how the efforts made in one research group might benefit other research areas, as well as highlighting the synergistic relationship between science and technology.
Betina da Silva Lopes, Portugal