Biomimetics: clingy as an octopus or slick as a lotus leaf? Teach article
Astrid Wonisch, Margit Delefant and Marlene Rau present two activities developed by the Austrian project ‘Naturwissenschaft und Technik zum Angreifen’ to investigate how technology is inspired by nature.

Image courtesy of chang /
iStockphoto
In 2004, Alice Pietsch from the University of Teacher Education Styriaw1, Austria, was inspired by a simple but very visual demonstration at a science museum. A retired science teacher was using bellows to pump air into a pair of sheep’s lungs, rhythmically inflating and deflating them. The crowd around him was much larger than around many of the more high-tech exhibits – which is what prompted Alice to create an interactive science museum.
In 2008, her dream came true. Over five months, Styrian students of all ages developed 50 different activities for other students. The exhibition Naturwissenschaft und Technik zum Angreifen (‘Science and technology to touch’) took place in 2009 at the Haus der Wissenschaft (‘House of science’) in Graz, Austria, where the students and their teachers helped the visitors with each activity. It was a huge success.
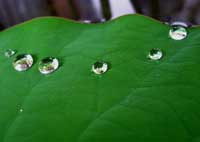
hydrophobic properties
Image courtesy of tanakawho;
image source: Flickr
Most of the activities from the exhibition are suitable for the science classroom; here we present two activities about biomimetics – the application of principles from nature to engineering and technology. Velcro, which mimicks the hooked seeds of burdock, and boat hulls that mimic the thick skin of dolphins are common examples. The activities below investigate suction pads and superhydrophobicity, both seen in nature. The activities were developed for younger secondary-school students (aged 10-15), but can easily be adapted for students of virtually any age and are a good opportunity for integrating physics, chemistry and biology. Depending on the level of detail you go into, the activities can each take anything from five minutes to over an hour.
The full collection of activities from the exhibition is available in print in German from Birgit Muhr (birgit.muhr@phst.at) for €19 plus postage.
Adhesion on flat surfaces: negative pressure
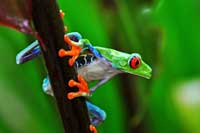
Image courtesy of Nickodemo;
image source: Flickr
Household suction pads were inspired by the soles of tree frogs’ feet and the tentacles of octopuses, whose suction power was already known to the ancient Greeks.
We use these pads on flat surfaces – to attach hooks to bathroom tiles or make kitchen machines more stable, and on rubber mats in the shower or toy arrows. But why do they stick?
If you look at a suction pad closely, you will notice that it is slightly curved. Could this curvature be important for the pad’s adhesive power? And why do you need to wet suction pads before using them? Let’s investigate.
Materials
- 4 suction pads (make sure you can attach a string to them)
- A small fretsaw
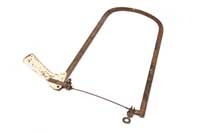
Image courtesy of
EddWestmacott / iStockphoto
- A 50 cm pole, about 3-4 cm in diameter (the students should be able to wrap their fingers around it easily)
- Four 50 cm pieces of strong string
- A pair of scissors
- A plastic board (about 1 x 1 m)
- A permanent marker pen
Procedure
- Using the fretsaw, make four equidistant grooves in the pole.
- Tie a piece of string to each of the four suction pads.
- Tie the other ends of the string to the four grooves on the pole. Make sure the four lengths of string are exactly the same length.
-
The setup for the adhesion
activity. Alternatively, a
single suction pad and a
shorter piece of wood can be
used (see back of the image)
Image courtesy of PHSt ArchivPut the plastic board on the ground, and use the permanent marker pen to draw four circles for the suction pads in a straight line. On either side of the line, draw a footprint (see image on right).
- Press the suction pads onto the four circles.
- What happens to the curvature of the suction pads when they are stuck to the plastic board?
- Stand on the footprints and try to pull the suction pads off the plastic by pulling on the pole.
- Repeat the experiment, wetting the suction pads before sticking them to the plastic board. What do you observe?
Discussion
When you press the suction pad onto the plastic board, you reduce the pad’s curvature, thereby reducing the volume of the space between the pad and the board, and causing some of the air between the pad and the board to escape. When you stop applying pressure, the elastic pad tends to resume its original, curved shape. The volume in the cavity between pad and board is increased again, but there is less air in it, and therefore also a lower air pressure. The higher pressure of the air outside the pad is what keeps the pad stuck to the board.
You can calculate the force of a suction pad as:
F = AP, where F: force; A: area; P: pressure.
The area will be πr2 where r is the radius of the pad. The pressure inside the cavity between pad and board is negligible when compared to atmospheric pressure, which is about 100 000 Pa. So:
F = πr2 (100 000 Pa)
The time it takes for the pad to come undone by itself (without applying additional force) will depend on how porous and flat both the rim of the pad and the underlying surface are, which determines how fast the air leaks back in and equilibrates the pressure.
Water, saliva and other liquids work well to seal the pad, making it more airtight and ensuring that air leaks back in less easily. Therefore, you need even more strength to pull the suction pads off if you moisten them before applying them to a surface.
Self-cleaning effects: hydrophobicity in nature

spotless even when
indulging in its favourite
pastime
Image courtesy of vendys /
iStockphoto
Nature has some real cleanliness fanatics: dung beetles crawl out of cowpats looking spotless, you rarely find a dirty butterfly or dragonfly, and some plants are very successful at shedding filth. The Indian lotus, for example, grows in muddy waters, yet no dirt clings to its leaves; in fact, in Buddhism, the lotus is a symbol of purity. You can find this lotus effect closer to home, too: nasturtium leaves and flowers look clean enough to eat, even without washing (rinse them anyway, just in case). How do they manage without soap?
Humans have replicated this self-cleaning effect for many purposes, for example, in self-cleaning plastics, roof tiles, windowpanes, ceramics, wood and car varnishes, and façade paint. You can also create dirt-repellent clothes by impregnating them with a special spray.
How does it work? Let’s find out.
Materials
- Parts of different plants with self-cleaning properties, such as nasturtium leaves (Tropaelum majus), lotus leaves (Nelumbo nucifera), cabbage leaves (Brassica oleracea), and tulip leaves (Tulipa spp.)
- Parts of different plants with hydrophilic leaves, such as leaves of the common beech (Fagus sylvatica) or the Southern magnolia (Magnolia grandiflora)
- Water, runny honey and / or liquid glue
- A Pasteur pipette and bulb, and some small spoons
- Ketchup, ground cinnamon and curry powder
- Newspaper, kitchen roll and textiles
- Impregnation spray (e.g. for waterproofing suede), self-cleaning nano-based façade paint, nano-coating for wood or glass
- Optional: a nano-sealed roof tile
Procedure
- Wet the self-cleaning plants with water, honey or glue and cover them with ketchup, cinnamon or curry powder (to simulate dirt). What do you observe?
- Repeat the experiment with the hydrophilic leaves. What do you observe?
- Now investigate the self-cleaning properties of artificial materials. Spray textiles, kitchen roll and newspaper with different types of nano-sealing treatments (e.g. impregnating spray, paint or nano-coating). Either compare the effect of the same type of treatment on different materials, or of different types of treatment on the same material.
- Use these and other nano-coated materials (e.g. tiles) to repeat the experiments, wetting and dirtying them. What do you observe?
Safety note
Some students may be allergic to some plants or parts of plants such as pollen. When using nano-coating, impregnation spray, etc., carefully read the instructions on the package. They may require the use of gloves or a well-ventilated space. See also the general safety note.
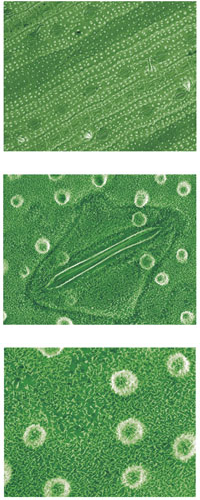
microscopy images at
magnifications of 300x
(top), 2500x (middle), and
3000x (bottom), showing the
wax structures on the
surface of a rice leaf, similar
to those on nasturtium or
lotus leaves. The first image
shows the waxy bumps
amongst the stomata; the
second image shows a stoma
close up; the third image
shows the papillate surface
of the leaf and the fine detail
of the wax structures
Images courtesy of Sarah
Perfect / Syngenta
Discussion
If you drop water or honey onto a lotus leaf, it will roll off very quickly. A close look under a scanning electron microscope reveals why: large numbers of tiny wax-covered bumps on the surface. These bumps are about 10-20 µm high and 10-15 µm apart from one another.
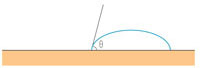
water droplet on a surface
Public domain image; image
source: Wikimedia Commons
How does this structure help the lotus leaf to remain clean? First, the surface of the lotus leaf is hydrophobic (water-repellent). The hydrophobicity of a surface can be quantified as the contact angle between the surface and the drop of water: the higher the contact angle, the more hydrophobic the surface (see image to the right). Surfaces with a contact angle <90° are called hydrophilic (‘water-loving’); those with a contact angle >90°, hydrophobic. Some plants, which are known as superhydrophobic, achieve contact angles of up to 160°, with only 2-3% of the drop’s surface in contact with the surface of the leaf. However, the lotus leaf is not only superhydrophobic but also covered in the aforementioned waxy bumps. These reduce the contact surface of the water droplet still further (imagine the drop sitting on the points of the bumps), with the result that the drop barely touches the leaf (only 0.6% surface contact) and runs off easily.
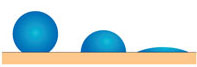
demonstratingdifferent levels
of hydrophobicity
Image courtesy of
Messer Woland; image source:
Wikimedia Commons
We have seen how the water flows off, but how do the leaves get rid of dirt particles? Plants are exposed to a variety of pollutants, many of which are inorganic (dust or soot), although others are of biological origin (e.g. fungal spores, conidia, honeydew or algae). On unwettable leaves such as those of the lotus, it is not only the adhesion of water to the surface that is reduced – dirt is also simply washed away by the water. This is not as obvious as it seems, though: there are two different types of dirt particles – hydrophilic and hydrophobic ones. Hydrophilic particles such as silt are taken up in the water droplet and cannot escape again. A track is visible on the leaf where the drops have taken up the particles and washed them away.
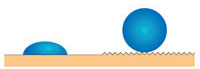
surface, a drop will be almost
spherical and have a very
low contact angle
Public domain image; image
source: Wikimedia Commons
But what about the hydrophobic particles? You might expect them to stick to the hydrophobic leaf surface, but in fact a drop of water will remove these particles too. How does this happen? The particles only touch the outermost tips of the wax structures, and since this contact surface is tiny, so are the adhesive forces between particle and leaf. They are so low that even the small adhesive forces between the water-repellent particle and water are stronger. So unlike the hydrophilic particle, which is taken into the drop, the hydrophobic particle sticks to the surface of the drop. The end effect is the same, though – it is washed off the plant.
Superhydrophobicity is not limited to lotus plants: other plants have self-cleaning properties thanks to mats of hair covering their leaves, butterfly wings have tiny ratchets that direct raindrops away from the insect’s body no matter whether the wing is tilted up or down, and the cuticle of dung beetles has embossed geometrical textures that make it hydrophobic.
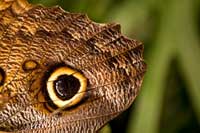
Image courtesy of Sebastian;
image source: Flickr
What is the advantage of these self-cleaning surfaces? For sedentary plants, they are a way of protecting themselves against micro-organisms, such as fungi, bacteria or algae. Most plants fight these enemies chemically through a large range of secondary metabolites, but preventing them from settling in the first place is potentially even more effective. In addition, if leaves are covered in dirt, this reduces the surface available for photosynthesis. The self-cleaning wings of butterflies have the advantage of not retaining water and becoming heavy, which would prevent the insects from flying.
Humans have developed many types of technology that emulate these hydrophobic effects. Impregnation sprays, for example, cover the material with a wax-like coating that makes the materials hydrophobic. Some façade paints go even further, forming tiny bumps when they dry. These bumps are as water-repellent as the lotus’s wax structures with the result that the painted surface becomes superhydrophobic.
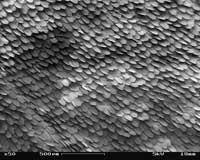
micrograph of the minute
scales that form the surface
of a Peacock butterfly wing
(magnification 50x)
Image courtesy of SecretDisc;
image source: Wikimedia
Commons
Acknowledgements
The two activities in this article were developed in the context of a workshop led by Astrid Wonisch for students of the Karl-Franzens-Universität Graz who were training to become biology teachers, and included in the 2008 exhibition Naturwissenschaft und Technik zum Angreifen.
Biology students Steffen Böhm and Karin Edlinger worked together with students from the combined 7th and 8th grade (aged 17-19) biomimetics class at the secondary school BG / BRG Petersgasse, Graz, a secondary school with a science and maths focus, and the school’s biology teachers, Renate Rovan and Ruth Unger, on ‘Adhesion on flat surfaces: negative pressure’.
Biology students Anna Freudenschuss and Ingo Fuchs worked together with students from a 4th grade (aged 14-15) class at the secondary school BG / BRG Fürstenfeld, Fürstenfeld, on ‘Self-cleaning effects: hydrophobicity in nature’.
Web References
- w1 – To learn more about the University of Teacher Education Styria, see: www.phst.at
Resources
- The German TV channel SWR has produced a series of short videos on suction pads in nature and technology, with accompanying teaching materials (in German). See their education website Planet Schule (www.planet-schule.de) or use the direct link: http://tinyurl.com/6gey6tg
- The following website offers a wealth of information on the lotus effect in English and German, including a small collection of videos: www.lotus-effekt.com
- For primary-school teaching activities on hydrophobicity and the surface tension of water, see:
- Kaiser A, Rau M (2010) LeSa21: primary-school science activities. Science in School 16: 45-49. www.scienceinschool.org/2010/issue16/lesa
- For a more in-depth overview of bio-inspired design, comparing the achievements of nature and technology, see:
- Vincent J (2007) Is traditional engineering the right system with which to manipulate our world? Science in School 4: 56-60. www.scienceinschool.org/2007/issue4/biomimetics
- For more information on biomimicry, see the Ask Nature website: www.asknature.org
- For further teaching activities on superhydrophobicity and nano-waterproofing for different age ranges, see:
- The EGFI website: http://teachers.egfi-k12.org/nano-waterproofing
- The Nanoyou website (http://nanoyou.eu) or use the direct link: http://tinyurl.com/6d88zmd
- The NanoEd resource portal (www.nanoed.org) or use the direct link to the PDF: http://tinyurl.com/6dwxo2b
- For more nanotechnology-related resources for the classroom, see:
- Harrison T (2006) Review of Nano: the Next Dimension and Nanotechnology. Science in School 1: 86. www.scienceinschool.org/2006/issue1/nano
- Hayes E (2010) School experiments at the nanoscale. Science in School 17: 34-40. www.scienceinschool.org/2010/issue17/nano
- Mallmann M (2008) Nanotechnology in school. Science in School 10: 70-75. www.scienceinschool.org/2008/issue10/nanotechnology
Review
Get stuck in! Or rather, give your students an opportunity to get stuck in with the activities described in the article.
The stickiness of tree frogs’ feet and of octopus tentacles prompts students to explore how this works using suction pads. Younger students will enjoy drawing around their feet and the noises that the suction pads make as they ‘slurp off’ when pulled. Older students can appreciate the physics and explain what is happening using calculations.
The stickiness of honey makes an appearance in the second activity, along with ketchup, cinnamon and curry powder – what student will not enjoy being let loose with these ingredients? Investigating non-stick lotus leaves and nasturtiums leads on to investigating novel self-cleaning liquids. This could lead to some interesting debates, as well as practical work that ranges from simple tasks for young students (rolling water off leaves) to much more complex work for older students, working with sprays and using scanning electron micrographs to explain phenomena.
The first activity covers physics (pressure) and the second activity, chemistry (surface interactions), both linking to biology with the idea that nature got there first – which could be taken further and investigated by the students. If different varieties of leaf are investigated, taxonomy can be introduced as well.
Here are some suggestions for questions you could ask your students after the activities:
- Draw a diagram to show a suction pad before and after it is pushed onto a surface. Annotate it to show areas of high, low and equal pressure.
- The article reminds you that force = area x pressure. Here this is used to calculate the force of a suction pad. Can you think of some other contexts in which this equation could be used?
- It is very annoying (and noisy) when a shower tray attached by suction pads to a tiled shower wall falls down. Explain what might make the suction pads ‘fail’ and cause the crash.
- The article mentions that “dung beetles crawl out of cowpats”. What might dung beetles be doing inside cowpats and why might they be crawling out? This might take some research!
- A scanning electron microscope is mentioned in the discussion of the second main activity. What is a scanning electron microscope? How does it differ from a transmission electron microscope?
- The bumps on a lotus leaf are up to 20 micrometres (µm) high. Express this height in a) nanometres (nm) and b) millimetres (mm).
- Explain, in your own words, what is meant by:
- Hydrophobic
- Hydrophilic
- Surface tension
- Pollutant
- Inorganic
You could also get your students to carry out a risk assessment for one or both of the activities. Some countries insist on these. Although it is the teacher’s role to provide a risk assessment, it is thought that if students are involved in creating their own risk assessments, they are likely to be much safer as they will have thought through the dangers themselves.
Sue Howarth, UK