Silken, stretchy and stronger than steel! Understand article
Could spider silk be the answer to medical and military challenges? Giovanna Cicognani from the Institut Laue-Langevin and Montserrat Capellas from the European Synchrotron Radiation Facility, France, investigate Christian Riekel and Tilo Seydel’s research into this remarkable material.
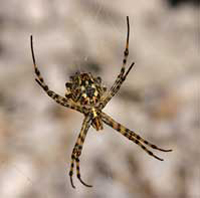
Davies
Biomimetics is a hot topic in modern science, but the idea that humans can exploit evolutionary designs is nothing new. More than 400 years ago, Leonardo da Vinci was studying birds to inspire his flying machines. Now scientists in France are using cutting-edge techniques to unravel the secrets of a material that has been around for more than 150 million years.
For centuries, we have envied spiders for their ability to create elegant webs. Although fragile in appearance, these webs can stop insects in mid-flight and are robust enough to restrain prey without the silken threads breaking. The threads which make up these remarkable structures are biopolymers. However, unlike man-made polymer fibres such as Kevlar®, which is made by forcing a hot and acidic polymer solution under pressure through small orifices (spinnerets) into a coagulation bath followed by washing, drawing and drying steps, silk is produced at ambient temperature and spun from aqueous solution. A spider-web’s ability to catch insects is due to the silk’s unique combination of mechanical properties: strength, extensibility (up to 30%) and, most importantly, toughness, or resistance to breakage. Spider silk may be six times stronger than steel by weight, but it is its toughness that makes it so special, as it allows it to absorb a large amount of energy without breaking. Man-made materials such as Kevlar are strong, but lack this specificity. Moreover, unlike Kevlar, spider silk is biodegradable and recyclable: when repairing their webs, spiders frequently eat damaged parts of the web and absorb the nutrients.
These special characteristics make spider silk of interest to many different research fields. A polymer based on spider silk could be used in medicine, as a high-strength, non-toxic suture, or in ligament repair, because the fibre not only does not tire when frequently flexed, but also can withstand regular impact and great pressure. The military sector is also investigating this material because its ability to dissipate energy could make it ideal for lightweight armour.
But before we can produce and use artificial spider silk, we need to understand what confers its unique mechanical properties. Recent experiments at the Institut Laue-Langevin (ILL) and the European Synchrotron Radiation Facility (ESRF) in Grenoble, France, have used neutron scattering and synchrotron radiation to investigate the microscopic characteristics of spider silk. This has provided researchers with a new insight into the silk’s structure, which in turn confers its mechanical properties. The two techniques, neutron scattering and synchrotron radiation, complement each other. Whereas synchrotron radiation, a type of very high-energy X-ray irradiationw1, enables a single silk fibre to be studied as it is extruded from a living spider, neutron scattering allows us to identify differences in the organisation of proteins and their accessibility to water, which has a strong influence on its mechanical properties. Neutrons, unlike synchrotron radiation, are scattered differently by normal water containing hydrogen and heavy water containing deuterium. By exposing a silk fibre to heavy water, we can determine from the way in which it scatters the neutrons fired at it which hydrogen atoms have been replaced with deuterium atoms. This in turn gives information about the chemical context in which the atoms are found.
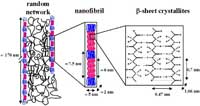
image to enlarge.
Image courtesy of Daniel
Sapede
The results, obtained by a joint ESRF and ILL team, in collaboration with the Department of Zoology at the University of Oxford, UK, showed that spider silk is a hierarchically organised material. Its composite biopolymer structure is made of proteins which are composed almost entirely of repetitive motifs formed by amino acids such as alanine and glycine. The alanine motifs form crystalline domains, which are separated by non-crystalline, glycine-rich domains.
These crystalline and non-crystalline domains are organised in nanofibrils, which are embedded in an amorphous protein matrix. Scientists are still debating how this structure results in the amazing mechanical properties of spider silk: is it due to ‘molecular springs’ in the amorphous protein matrix or to the properties of an amorphous network, reinforced by crystalline domains.
Scientists have been able to artificially produce the spider silk proteins themselves for some time, and we now understand in greater, although incomplete, detail how the proteins are organised to confer the impressive toughness of spider silk. Nonetheless, further work is necessary to understand – and replicate – the mechanism of protein aggregation and fibre formation. In the spider, the silk proteins are synthesised and secreted in a gland as a kind of a viscous liquid crystal. This liquid is then pushed through a long duct to a spigot at the end of the spider’s spinneret. On its way out, a thickening process and pH change alter the viscous liquid, resulting in the aggregation of the silk proteins. The spider is even capable of resorbing and recycling water during the thickening process. Other factors, such as the movement of the spider’s body, also play an important role in the spinning process.
Exactly how these factors interact, and how they could be mimicked to produce artificial spider silk in laboratories, is a question that continues to occupy scientists. At ESRF, ILL and other institutes around the world, biomimetic research continues into a man-made alternative to one of the most remarkable natural materials – and with it, a new generation of cheaper and more ecological materials.
Web References
- w1 – For more details of how synchrotron radiation is used in research, see: Capellas M, Cornuéjols D (2006) Shipwreck: science to the rescue! Science in School 1: 26-29.
Resources
- ESRF (2006) Nature inspires technology. ESRF Newsletter, Issue 43, June.
- Forbes P (2005) The Gecko’s Foot. London, UK: Fourth Estate
- Sapede D (2006) Contributions à la compréhension de la structure et de la dynamique hiérarchiques du fil de traîne de l’araignée. PhD thesis. Grenoble, France: Université Joseph Fourier.
- Vincent JFV (2007) Is traditional engineering the right system with which to manipulate our world? Science in School 4: 56-60.
Institutions
Review
This article could be used in chemistry, biology and physics lessons, for children aged 11-17. In particular, it is applicable to considerations of crystallography, lattice structures and the role of pH in protein aggregation.
Eric Demoncheaux, UK