Fusion – ace in the energy pack? Understand article
The energy demands of our society continue to increase, while the stocks of fossil fuels - still our major energy source - are declining. Chris Warrick from the European Fusion Development Agreement explains why research into fusion offers the hope of a safe and environmentally responsible energy…
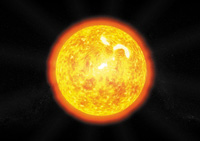
on earth the kinds of
reactions observed in the sun
Harnessing fusion – the power source of the sun – has long been a dream, as it could provide an almost limitless supply of energy in an environmentally responsible way. With the construction of a new reactor-scale fusion device, ITER, about to start in Cadarache in the south of France, that dream will come a large step closer to reality.
Why research a new form of energy production? Worldwide energy use is expected to double in the next 40 years, and lifting developing countries out of poverty will require an even larger increase. At present, 80% of the world’s energy is generated by burning fossil fuels – driving global and climate change and generating pollution. Eventually, fossil fuels will be exhausted; the first sign will be a decrease in the rate at which oil can be produced, which could occur relatively soon. There is no magic wand that will solve the problem. Instead, a measured response to the problems we face would include a cocktail of approaches. Likely ingredients will be an increase in energy efficiency, a deployment of more renewable and nuclear fission energy sources, and intensified research into new technologies such as fusion.
The idea of extracting the net energy from controlled fusion reactions is nothing new. The difficulty has been recreating on earth the kind of reactions observed in the sun. Unlike fission (splitting apart) of heavy nuclei, fusion of light nuclei requires extreme conditions to be attained and sustained. The chosen reaction in experiments here on earth is the fusion of deuterium and tritium – two heavy forms of hydrogen – producing helium and a neutron, which carries the excess energy. This requires a high temperature (or energy) of the particles in a super-hot ionised gas called a plasma: around 150 million degrees Celsius. This must be combined with a sufficient density of particles and a confinement method that enables the plasma energy to be held inside the plasma long enough for the fusion reaction to become established and for more power to be released than is needed to keep the fuel hot.
One important feature of fusion energy, and one which distinguishes it from fission, is that fusion is not a chain reaction. This makes it inherently safe: there is no possibility of a ‘runaway’ reaction. At any one moment, only a few grams of fuel are present in the plasma vessel, enough for a minute of burn time. To stop the reaction, it suffices to stop the supply of fuel, just like in a gas oven.
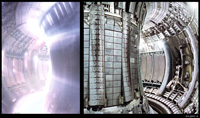
with plasma inside (left)
For the past three decades, the strongest line of research has concentrated on the so-called tokamak device. In a tokamak, a doughnut-shaped ring of plasma is confined in a vacuum vessel. To heat the plasma to 150 million degrees Celsius, an effective way of holding the plasma away from the vessel walls is needed. As the plasma comprises charged particles (ions and electrons), this is done with powerful magnetic fields generated by large magnetic field coils around the vessel.
Heating the plasma to such extreme temperatures requires several complementary methods. One of these methods consists of passing a powerful electrical current through the plasma, while additional heating power is provided by externally injected microwaves and beams of neutral particles.
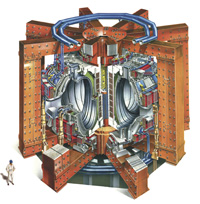
Significant progress has been made in tokamak experiments all around the world, with the European-funded Joint European Tokamak (JET) device at Culham Science Centre in the UK providing the focus of the European research effort. JET is at the pinnacle of European fusion research, and is supported by many smaller tokamaks at laboratories across the continent.
The programme of work on JET is organised and co-ordinated by the European Fusion Development Agreement. As the largest operating tokamak in the world and the only one capable of operating with both fusion fuels (deuterium and tritium), JET has obtained the necessary conditions in the plasma for fusion to occur. More crucially, JET has provided valuable lessons in how to maintain plasma stability and confinement in the face of a host of plasma instabilities.
The measured fusion output power in JET (approximately 16 megawatts) is close to, but still lower than, the power required to maintain the plasma at fusion temperatures (25 megawatts). Less power out than in – not promising for a power station! However, scaling studies using data from JET and smaller fusion experiments tell us that a larger machine will reverse this balance, producing a much larger fusion output power than the power needed to heat the plasma.
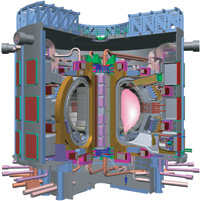
Although there is confidence that a fusion power station can be constructed in this way, further studies are required, especially into the technologies needed to ensure that a fusion power plant is safe, reliable and economically viable. The International Tokamak Experimental Reactor (ITER) will be such a device: a stepping-stone to a commercial fusion power station. It will be twice the size of JET, and should produce over 500 megawatts of fusion power in ten minutes – at least ten times the power needed to heat the plasma.
As its name suggests, ITER is an international project with seven partner organisations: the EU, Japan, USA, South Korea, China, Russia and India. The design – using cutting-edge technologies such as superconducting magnetic field coils and advanced instrumentation – is already finished and a decision was taken in June 2005 to build the device at Cadarache in the South of France. Preparations are now underway to start construction, ready for operations to commence in 2015.
One issue that inevitably arises with this technology is the use of radioactive tritium. In a future fusion power plant, tritium will be made inside the reactor itself in a closed cycle, so no transport of radioactive material outside the plant will be necessary during operation, and only a limited quantity will be present in the plant. The inside of the plasma vessel will become radioactive during the operation of the plant due to the neutrons produced in the fusion process. However, if the appropriate materials are used, these can either be disposed of as non-radioactive waste or recycled after a period of about 100 years, owing to their relatively short half-life. This compares favourably with the many thousands of years required before the components of a fission reactor become safe to handle. Part of the fusion research programme focuses on developing these ‘low-activation’ materials.
ITER is essential for testing and integrating the various technologies on the scale of a power station, and should confirm that it is possible to build a fusion power station. However, before a fusion power station can be built, years of testing of the materials under working conditions will be required before a licence is granted, to ensure that it is safe and reliable. This can only be done in a special device called the International Fusion Materials Irradiation Facility (IFMIF). ITER, which will cost some €5 billion, and IFMIF, which will cost slightly under €1 billion, are key to the development of fusion as a workable energy source. Although these costs may sound high, they are very small on the scale of the total world energy market, approximately €3 trillion per year.
So, the big question is ‘when will fusion produce electricity for the world?’ Building and operating ITER and IFMIF in parallel should allow the first prototype fusion power station to produce power in 30 years’ time. Although this is not soon enough for an imminent move away from fossil fuel energy sources, it will enable fusion to provide a very valuable extra energy option for the latter half of the 21st century.
EFDA education and outreach
Many of the fusion research institutes in the European Fusion Development Agreement (EFDA) have their own outreach programmes, which often include lectures, and visits to schools and research facilities such as JET. Details of the individual research institutes are available on the EFDA website.
Within the framework of the EIROforum, EFDA participates in Science in School, the Science on Stage festival and other outreach and education projects.
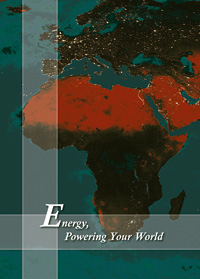
schools
EFDA has produced a 60-page brochure for secondary schools, ‘Energy, Powering Your World’, giving a broad introduction to the world of energy. Topics include the ways we use energy in our daily lives, where it comes from, and how we will deal with our energy needs in the future. Suggestions for energy discussions in the classroom are available as a separate, accompanying document.
To receive a free printed copy of the brochure, available in English and Dutch, send an email to aline.duermaier@efda.org, including your name, postal address and the number of copies you would like (up to five). The brochure can also be downloaded in electronic form (including in in Spanish, French, German and Italian) from the EFDA website.
EFDA has a range of other educational materials available, such as a CD-ROM, ‘Fusion, an energy option for the future’, and a general poster on fusion, both of which can be requested via the EFDA website. The website also provides basic and more advanced information about fusion science.