Fantastic feats: experimenting with water Teach article
How can air hold the water in an upturned glass? Why does water stay in a bottle with a hole in its base? Find out with these entertaining experiments.
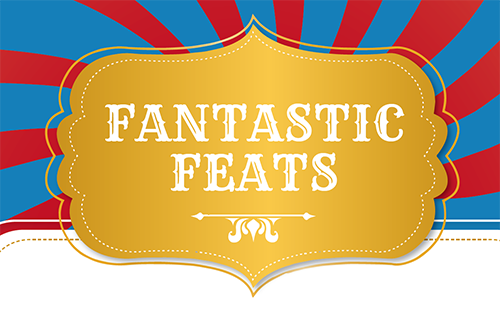
From their earliest years, children enjoy playing with water, and so do many older students. In this set of experiments, we look at the forces that are significant when dealing with water, demonstrating some basic science principles – and some surprising results. All the experiments are safe to do at home as well as at school, and require just simple household objects as the equipment, plus plenty of water.
Experiment 1: the upside-down glass
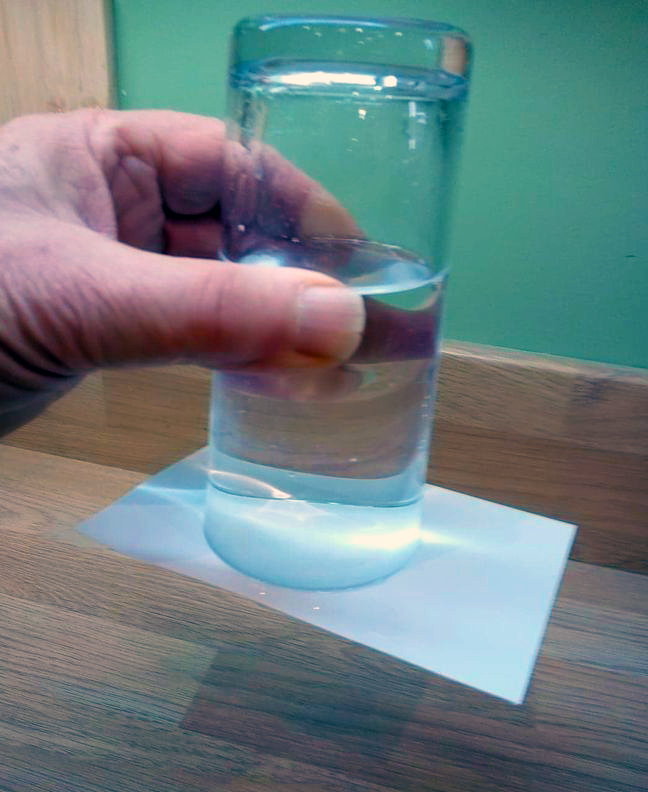
turned upside down. Why
does the card stay in place?
Image Courtesy of David Featonby
Many people have tried this experiment in some version, but can you work out what’s really going on?
Materials
- Straight-sided water glass
- Piece of thin card (large enough to cover the open end of the glass)
Procedure
- Pour water into the glass until it is nearly full.
- Place the piece of card on top of the beaker.
- Turn the beaker upside down with one hand, holding the card in place with the other hand.
- Remove the hand holding the card (figure 1).
- Note what happens. Does the card fall off and the water fall out? Can you explain why not?
Discussion
Surprisingly, when the glass is inverted, the card and the water remain in place. Why is this?
Let’s consider the forces on the card. These are:
- gravity, from the weight of the card itself (acting downwards)
- gravity, from the weight of the water pushing on the card (acting downwards)
- air pressure, which pushes on the outer surface of the glass and card, acting at 900 to the surface of the card (so producing an upward force on the card where this has just water above it).
So in this experiment, the force of air pressure pushes upwards on the card at the open end of the glass, opposing the force of gravity and keeping the water in the glass.
Extension: estimating the upward and downward forces
How do we know that the upward force of air pressure is enough to oppose the downward force gravity, to hold the water in the glass? We can estimate these forces quite easily.
The weight of the card is much less than that of the water, so to simplify we can ignore the weight of the card itself. This means that the downward gravitational force on the card is the weight of the water column
= h x A x ρ x g
where h is the height of the water column, A is the cross-sectional area of the glass, ρ is the density of water (1 000 kg/m3) and g is the acceleration due to gravity (approximately 10 m/s2).
So if h is 10 cm (0.1 m) and A is approximately 25 cm2 (0.0025 m2), the downward force is approximately
0.1 x 0.0025 x 1000 x 10 = 2.5 N
For the upward force, this is the atmospheric pressure, P, multiplied by the area over which it acts, A.
Atmospheric pressure is approximately 100 000 Pa (pascals, or N/m2).
So the upward force on card = P x A
= 100 000 x 0.0025 = 250 N
So for a 10 cm water column, the upward force due to the atmosphere on the card far exceeds the downward force of gravity on the card due to the water.
We also need to recognize that the air above the water plays a significant role. If this remained at atmospheric pressure, the weight of the water would be sufficient to remove the card, however as soon as the water exerts a downward pressure on the card, it reduces the pressure of this trapped air, which is sufficient to enable the upward atmospheric pressure on the card to support the water. A 1/100 change in volume of this air is sufficient to balance the water, i.e., the pressure reduces by 1/100th which is equivalent to the pressure of the water.
Further investigation
You can also think about the questions below, and perhaps carry out further experiments to answer some of them:
- Does this experiment work if the glass is completely full of water?
- How does the ratio of air to water change the experiment outcome?
- Would this experiment still work, no matter how tall the glass is?
- What other shaped containers (e.g., bottles) can be used?
Experiment 2: water’s invisible ‘skin’
In this experiment, we discover how cohesive forces within water act like an invisible ‘skin’ that can keep the liquid in an upturned cup – sometimes.
Materials
- Paper cup
- Piece of thin woven nylon cloth (large enough to cover the open end of the cup)
- Elastic band
- Thin card (large enough to cover the open end of the cup)
Procedure
- Cover the open end of the cup with the nylon cloth (figure 2, left).
- Pull the cloth tight, and secure it with the elastic band (or glue it to the cup around the rim).
- Pour water into the cup through the cloth, nearly filling it.
- Place the card over the nylon and the open end of the cup.
- Turn the cup upside down.
- Note what happens: the water should stay in the cup, as in experiment 1.
- Now carefully remove the card. Does the water flow out through the nylon cloth? If not, why? Water was poured in through the cloth, so why doesn’t it pour out again?
- To pour the water out turn the cup upright again quickly, then tip up the cup slowly while pressing a finger on the nylon (figure 2, right).
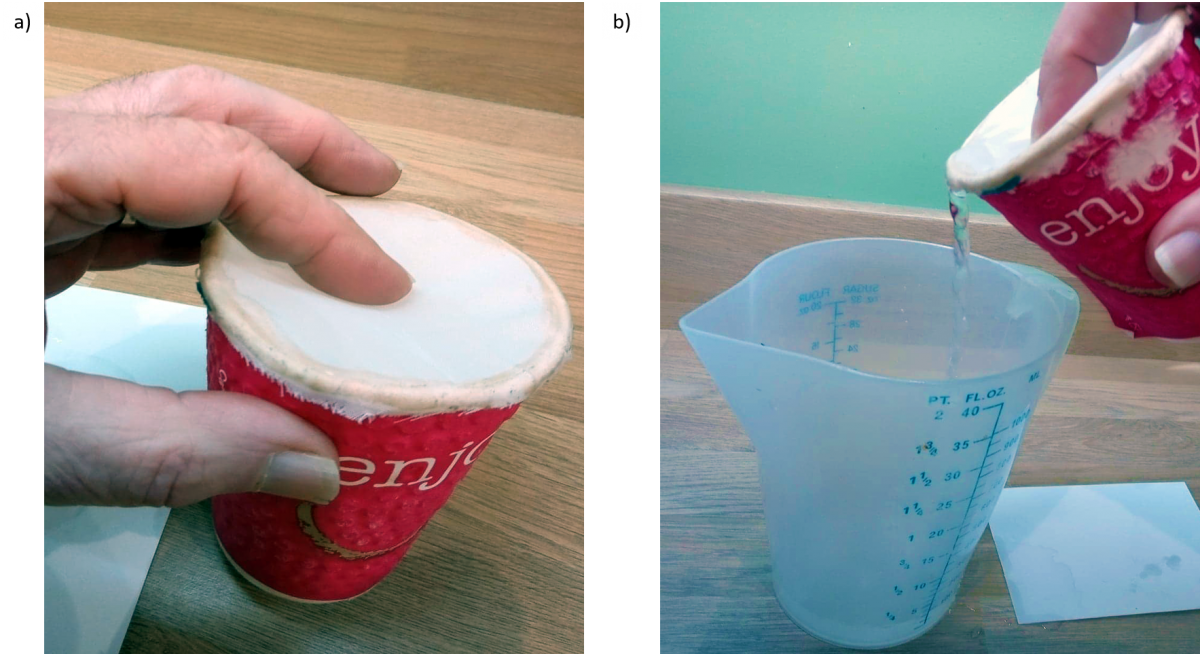
Image Courtesy of David Featonby
Discussion
The reason why the water does not flow out through the very small holes in the nylon is because there are forces of cohesion between the molecules in the water. These forces make the surface of the water act like a ‘skin’ between the tiny holes in the nylon cloth. This effect is known as surface tension, and it is the same principle that keeps you dry under a woven nylon umbrella: there are tiny holes in the cloth, but the rain won’t get through due to the cohesive forces of surface tension between water molecules.
Further investigations
There are plenty more experiments you can do with surface tension and molecular cohesion. Perhaps look up ‘surface tension experiments’ on the internet and see what other activities you can find?
Here are two further simple experiments you can try.
Paperclip boat
Take a dish of clean water and a paper clip. Hold the paperclip in a strip of paper towel and lower it into the water. Then allow the paper to sink or carefully sink it with a toothpick. The paperclip will appear to float but is in fact being held by the surface tension of the water.
What else can you ‘float’ – for example, a ring pull from a drinks can? What happens to the paper clip if a drop of washing-up liquid is added to the water? How can you explain what you see?
This effect can also be used to make a ‘soap boat’.
Joined-up water jets
Take a clean empty drinks can, plastic cup, or bottle and make three small holes close together, near the base. Fill the can with water, and when three jets come out, use your fingers to try and join the jets together. You will be able to do this, because of the cohesive forces between water molecules.
Another fun experiment to illustrate cohesion is pouring water down a string.
Experiment 3: bottled water
These activities use a simple bottle of water to reveal some surprising effects due to surface tension and gravity. It’s a good idea to do them outdoors because water spillage is likely (see figure 3).
Materials
- Plastic bottle (250 ml) with screw cap
- Large needle or nail
Procedure
Use the needle or nail to make one (or more) very small holes near the base of the plastic bottle by heating it over a flame (safely held) until it is hot enough to melt the plastic. In schools, this should be done by the teacher in advance of the experiment, for safely.
- For fun, you can add a label to the bottle saying ‘Do Not Open’ – and see if people ignore this.
- With the cap off, quickly fill the bottle with water, holding your finger over the hole, and then replace the cap.
- Hold the bottle still (or hand it to someone else) with the cap closed. What happens to the water?
- If the warning is ignored and the bottle cap is opened, what happens?
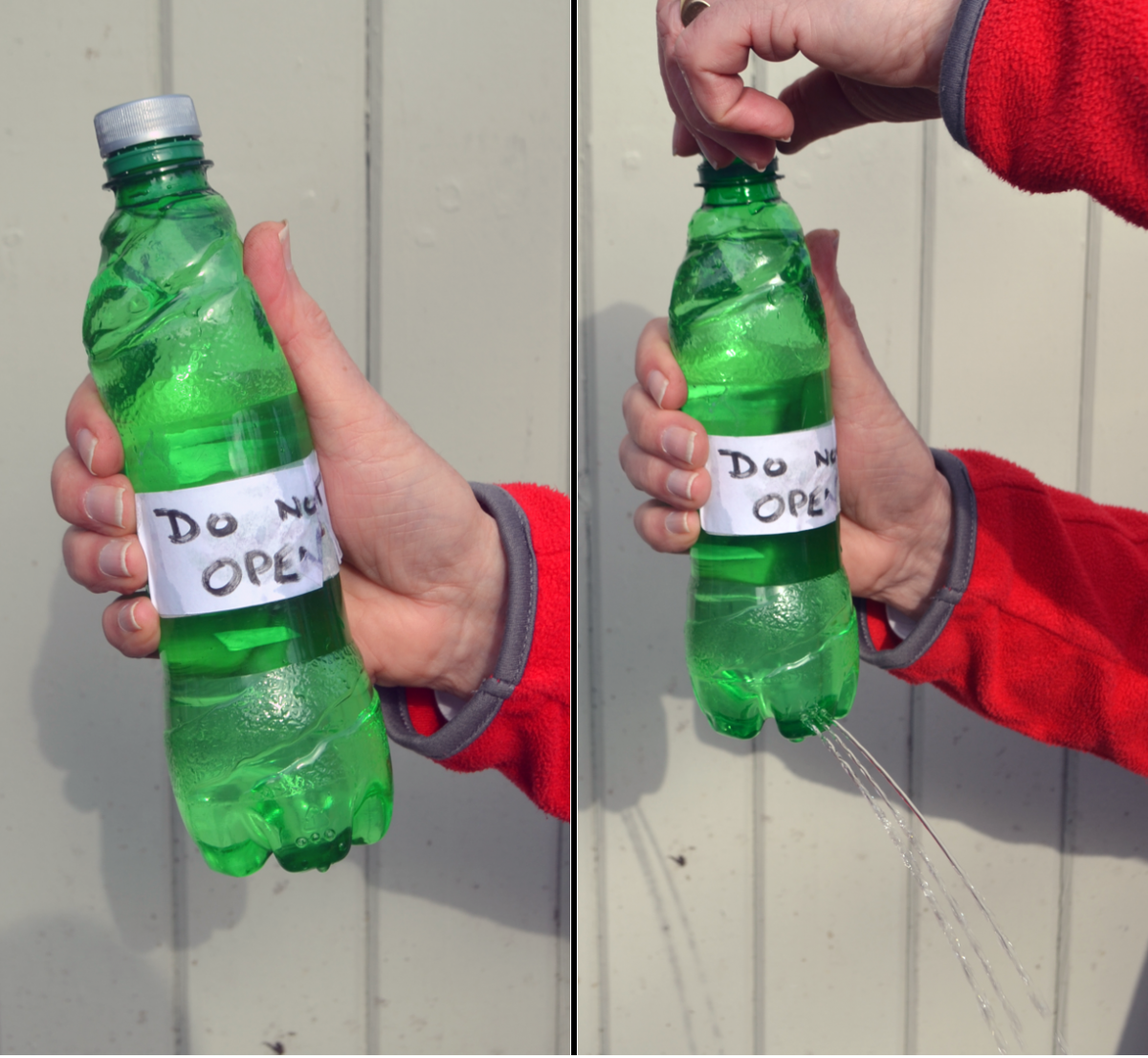
Image Courtesy of David Featonby
Discussion
Once a container is sealed, water will only flow out of a small hole if that water can be replaced by air or more water. A bottle with one small hole can therefore hold water if the cap is sealed. Once the cap is unscrewed the water will flow out, due to the weight of the water. The hole needs to be small enough for the surface forces to hold the water.
Further investigations
Another interesting experiment to try with a full bottle of water with a hole near the base is: what happens when you throw the bottle up and catch it?
If you fill the bottle with water and hold it with the cap unscrewed for a few seconds (figure 4), the water will flow out of the hole.
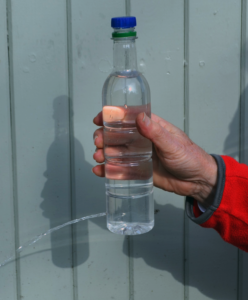
Image Courtesy of David Featonby
Now throw the bottle up in the air (figure 5), and watch it carefully as it falls. Observe each part of its journey – on the way up, at the top of its flight, and on the way down.
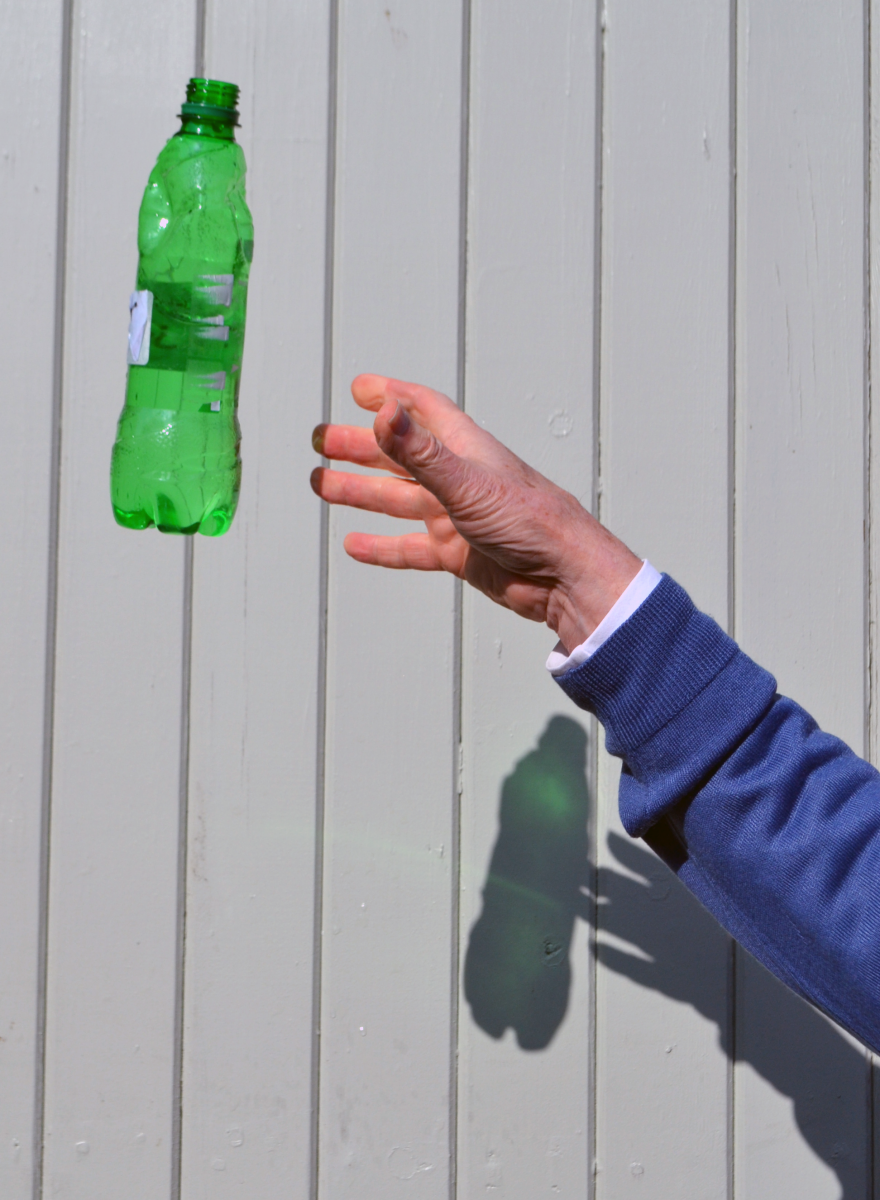
Image Courtesy of David Featonby
When the water is in free fall (i.e. on the way down), water will cease to flow out of the bottle. This is because the water within the bottle becomes weightless relative to the bottle itself, as both the bottle and its contents are in free fall. Thus, in this situation the weight of the water does not force it out of the bottle.
This effect can also be demonstrated with a water-filled hollow tube (around 50 cm, with a diameter that can easily be covered by a finger). The bottom of the tube is covered with one hand while it is launched into the air, with this hand exerting the launching force and the other just supporting the tube. However, this can be a little tricky to demonstrate because it is essential that the hand covering the tube end is the last to let go when throwing the tube up and the first to contact the tube again on catching it, to avoid accelerating/decelerating the tube without the water column.
You can also try to answer these final questions:
- What happens to the water when the bottle is travelling upwards during the throw? Can you explain this?
- If you try to catch the bottle, what happens to the water? Can you explain this?
- What else can you throw in the air so that there is a change in what happens when it is in free fall, compared with when it is stationary? Hint: Think of toys or devices that work with gravity, e.g. where particles or moving parts or liquid fall through a gap.
Resources
- A more detailed version of the thrown water bottle experiment: Tsakmaki P, Koumaras P (2017) When things don’t fall: the counter-intuitive physics of balanced forces. Science in School 39:36–39
- Try a similar experiment to the paper cup and nylon cloth activity: https://www.stevespanglerscience.com/lab/experiments/water-screen/
- Watch this video with more activities to try with your students using water: https://www.youtube.com/watch?v=CCxbI1qRsWY&ab_channel=DrewtheScienceDude
- Learn how to make a soap boat: https://www.youtube.com/watch?v=OU76wwmg9Hs
- Watch a video on the running water experiment: https://www.youtube.com/watch?v=8nOU7jbRPPo&ab_channel=DrBoydTheChemist
- Read other Teach articles from the Fantastic Feats series:
- Featonby D (2017) Fantastic feats. Science in School 39:45–47
- Featonby D (2018) Further fantastic feats: falling and bouncing. Science in School 43:37–54
- Featonby D (2019) Fantastic feats: magic with money. Science in School 47:46–50
Review
The simple experiences shown in the article, easy to reproduce and using materials that are easy to find, allow students to approach the topics of surface tension and pressure in liquids.
The author, also, by highlighting the “magic side” of some experiments, makes them more interesting and also suitable for the general public.
The article offers the possibility to make interdisciplinary links to biology topics, such as pulmonary respiration (and how nature provides the alveoli with surfactants to decrease surface tension),
capillarity in plants, or how the surface tension allows some insects to walk on the surface of the water
Maria Teresa Gallo, Math and science teacher, Italy