Destination fusion Inspire article
Five young researchers are working to create a star on Earth.
What does it take to become a fusion researcher? A fascinating and multidisciplinary field, fusion needs people with a variety of expertise and skills. From physics and material sciences to engineering and management, there’s a lot that goes into getting a fusion experiment running.
Fusion is the process that powers stars, including our Sun. To replicate the fusion process on Earth, researchers have to use heavy hydrogen isotopes – deuterium, which is readily available from water, and tritium – and temperatures almost six times hotter than the core of the Sun. It may be fair to say that generating energy from fusion is one of the greatest challenges for humankind. But fusion researchers have accepted this challenge because, once harnessed, fusion provides nearly unlimited, safe and clean energy.
EUROfusion, the consortium of fusion research institutes across the European Union and Switzerland, promotes education and training in fusion by supporting young fusion researchers. Talking to holders of EUROfusion grants can give us a glimpse into the world of fusion research. For Llion Marc Evans, Mélanie Preynas, Gergely (Geri) Papp, Mike Dunne and Matthias Willensdorfer, it is about more than just physics and engineering. It is the excitement of working in a multidisciplinary field, the drive to see fusion power light up homes across the globe, and the thrill of being part of a future energy solution.
Llion Marc Evans
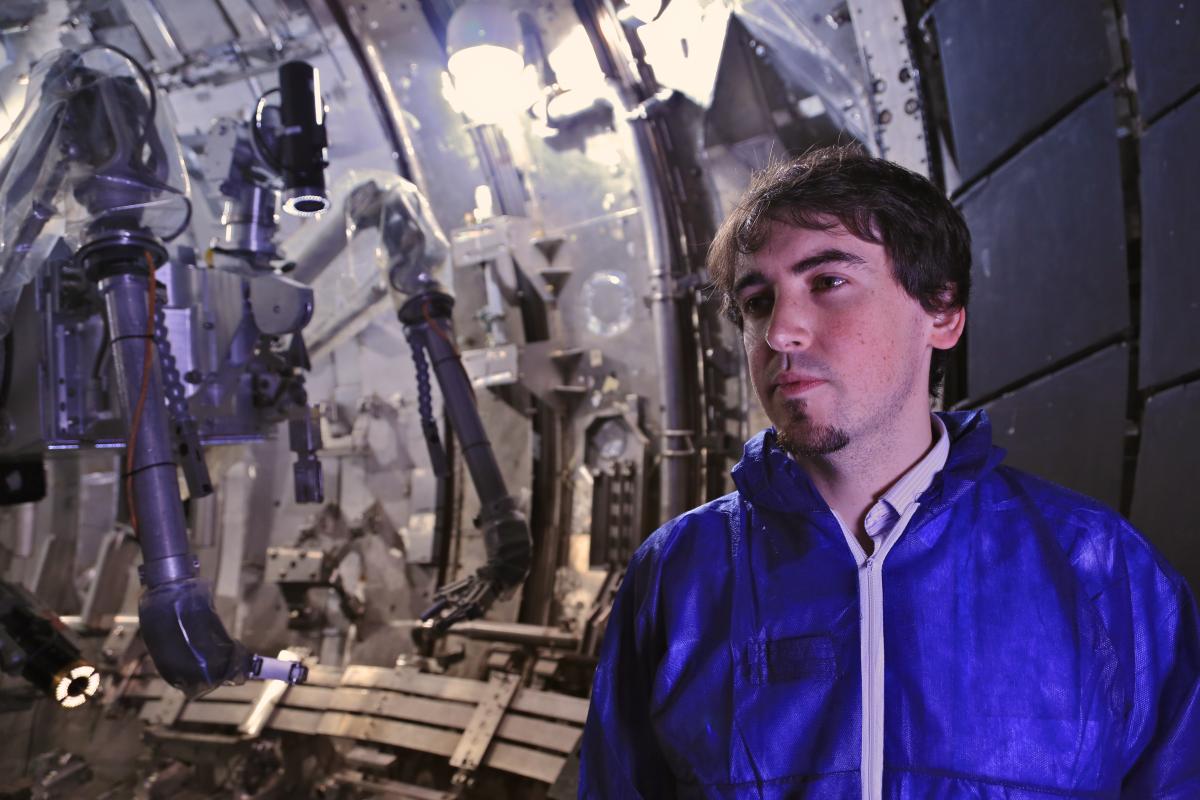
European Torus.
Image courtesy of Haydn
Denman
Armed with a masters degree in aeronautics, Welsh physicist Llion Marc Evans was on track for a career in the fast-paced world of Formula 1 racing before he made a pit-stop. After hearing stories about sustainable energy and fusion power, Llion changed tracks and drove straight into fusion research.
Back in 2008, when Llion was considering his options for a PhD, reports about climate change kept taking his interest. “I watched a BBC Horizon episode on fusion energy, which convinced me that research in fusion would be exciting and that I could contribute towards fighting climate change,” he says.
In November 2014, after Llion completed his PhD on materials used in fusion reactors, EUROfusion awarded him a Researcher Grant, and in August 2015, Llion began working as the EUROfusion Fellow at the Culham Centre for Fusion Energy in Oxfordshire, UK. Currently, he is working on a method to convert 3D X-ray images of components for the planned demonstration power plant DEMO into high-resolution computer models. “By creating a ‘digital twin’ you model real parts instead of idealised designs,” he explains. “Achieving better accuracy helps the design process and improves our predictions of the power plant’s performance.”
The blurred lines between physics, engineering and materials science, and foundations in experimental, computational and theoretical fields are what makes fusion research exciting for Llion. Other than many cups of coffee, he says, nothing in the day of a fusion researcher is typical. “One day, I might be using a facility such as the neutron source ISIS, another day it might be one of the world’s most powerful computers like ARCHER or I may just be sitting in the library reading journal papers,” he adds.
But ultimately, it is the potential that fusion research has to bring about a positive change at a global scale that is most appealing to Llion. “My hope is that I’ll see the first fusion power plant put electricity onto the grid before I retire and that I’ll have played a part in that moment.”
Mélanie Preynas
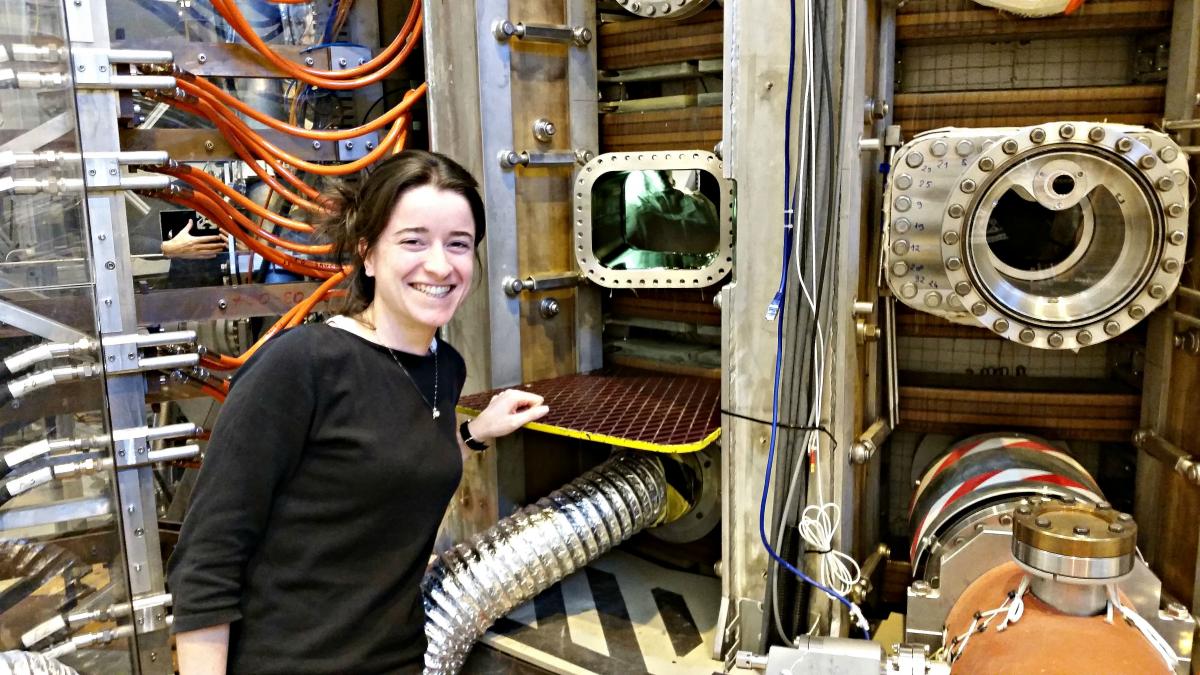
tokamak of the Swiss Plasma
Center.
Image courtesy of Olivier
Sauter
When it comes to states of matter, the fourth state, plasma, is not as widely known as solids, liquids and gases. However, it is the most abundant form of matter in the Universe, and is crucial to nuclear fusion. One ingredient for a successful fusion experiment in a tokamak, currently the most advanced fusion device, is a well-behaved and stable plasma, but achieving good behaviour and stability is not trivial. This challenge is one of the things that physicist Mélanie Preynas tackles. Her work aims to control, in real time, what are known as ‘magnetohydrodynamic’ instabilities in plasma.
Simply put, magnetohydrodynamics refers to studying the magnetic properties of electrically conducting fields such as plasma. Magnetic fields induce currents in the conductive fluid, which in turn modifies the geometry and strength of the magnetic fields themselves. “To achieve stability and good performance of the plasma, we have to control it in real time,” says Mélanie. “One possibility is to launch microwaves into the vessel of the tokamak to generate heat and drive the current,” she explains.
Mélanie, who is currently working at the Swiss Plasma Center of the École Polytechnique Fédérale de Lausanne, discovered the world of fusion research during her PhD at the Institute of Magnetic Fusion Research in France. The idea of developing a new energy source is what initially drew Mélanie to fusion research. Once in, she was excited by the multidisciplinary nature of this field. “It’s a mix of theoreticians and experimentalists working with technicians and engineers to obtain and understand experimental results of the fusion device,” she says.
The life of a young researcher in fusion can be exciting, fun and competitive. Mélanie’s work is also varied, and about more than just working on tokamaks. “I may spend time in my office analysing experimental data, or an entire day in the control room of the fusion device during an experimental campaign or making some calibration of a measurement system on the machine,” she points out. Mélanie is looking ahead and hopes to continue working for fusion research in the future.
Gergely (Geri) Papp
Image courtesy of EUROfusion
How far can childhood fantasies take you? In the case of EUROfusion fellow Gergely (Geri) Papp, they paved the way for his career as a fusion researcher. Geri, who was (and still is) a great fan of science fiction, learnt that there is nothing fictional about the fusion reactors that he came across so often in the pages of science fiction. “Part of the reason why I became a physicist is that I wanted to work with fusion,” says Geri, who initially studied physics in his home country of Hungary before completing his PhD.
Geri currently works at the Max Planck Institute for Plasma Physics in Garching, Germany, which houses the tokamak ASDEX Upgrade. “It’s a fantastic machine to work with because it allows you to plan and execute experiments on a relatively shorter time-scale as compared to larger experiments, and at the same time gives results that are crucial,” he explains.
One of the projects that Geri works on involves runaway electrons. Within the fusion reactor a mixture of hot electrons and positive ions moves in random directions. Most particles move around harmlessly, colliding with each other, but some fast-moving electrons, known as runaway electrons, collide with the walls of the fusion experiment vessel. “Our goal is to better understand the formation process of such electrons and to design a safety system to eliminate or mitigate them,” Geri explains.
From carrying out experiments to developing theoretical models, implementing numerical codes, teaching, publishing scientific papers and giving talks, the life of a fusion researcher is exciting. But the ultimate motivator for Geri is the vision of realising fusion energy. “When achieved, fusion will be as close as possible to an ideal energy source: clean, safe, abundant, accessible and controllable.” The fact that power from fusion might be decades away does little to dampen Geri’s enthusiasm. “It took humankind more than 6000 years to learn how to fly or build computers; even if it takes an extra 50 years to realise fusion power, I can live with that.”
Matthias Willensdorfer
ASDEX Upgrade.
Image courtesy of EUROfusion
When experiments are running at ASDEX Upgrade, you can find Matthias Willensdorfer in the control room. Sitting between 30 other scientists, his task is to ensure that the electron temperatures measured inside the tokamak, the device responsible for producing controlled fusion reactions, are correct.
Whenever there is a failure in the complex setup of his diagnostics, Matthias has to rush to resolve it. It could be a power supply breaking down in one of the components or a device deep in the signal chain not working correctly. And he has all of twenty minutes to do this before the next cycle of the experiment starts. “It can be exciting… and stressful,” he says with a smile.
Matthias joined the Max Planck Institute for Plasma Physics in Garching, Germany, as a EUROfusion fellow after completing his PhD and working as a guest scientist at ASDEX Upgrade. He says that running experiments is just one facet of his job. “You can spend anything from a few months to a few years developing and building new diagnostics or planning new experiments,” Matthias explains. Experimental work and the anticipation of the results make fusion research exciting for him. “Fusion science combines several fields such as fluid dynamics, electrodynamics and plasma physics… it never gets boring.”
By delving into physics, data analysis and programming, Matthias is striving to understand the response of the plasma to external magnetic perturbations produced by additional coils. Fusion plasmas can develop various kinds of instabilities and some of them, such as the edge localised modes (ELMs), have the potential to damage the inner lining of the wall of a tokamak. “External magnetic perturbations, though small, can be used to mitigate such ELMs,” explains Matthias. “In theory, the plasma can weaken or amplify these perturbations and can therefore change the behaviour of these edge instabilities. My task is to measure and characterise this response and compare it with different theories.”
The experiments, data analysis and programming aside, Matthias says that there was also some idealism behind his decision to pursue fusion research. “The energy problem is huge, and I want to do something about it.” And fusion research it was.
Mike Dunne
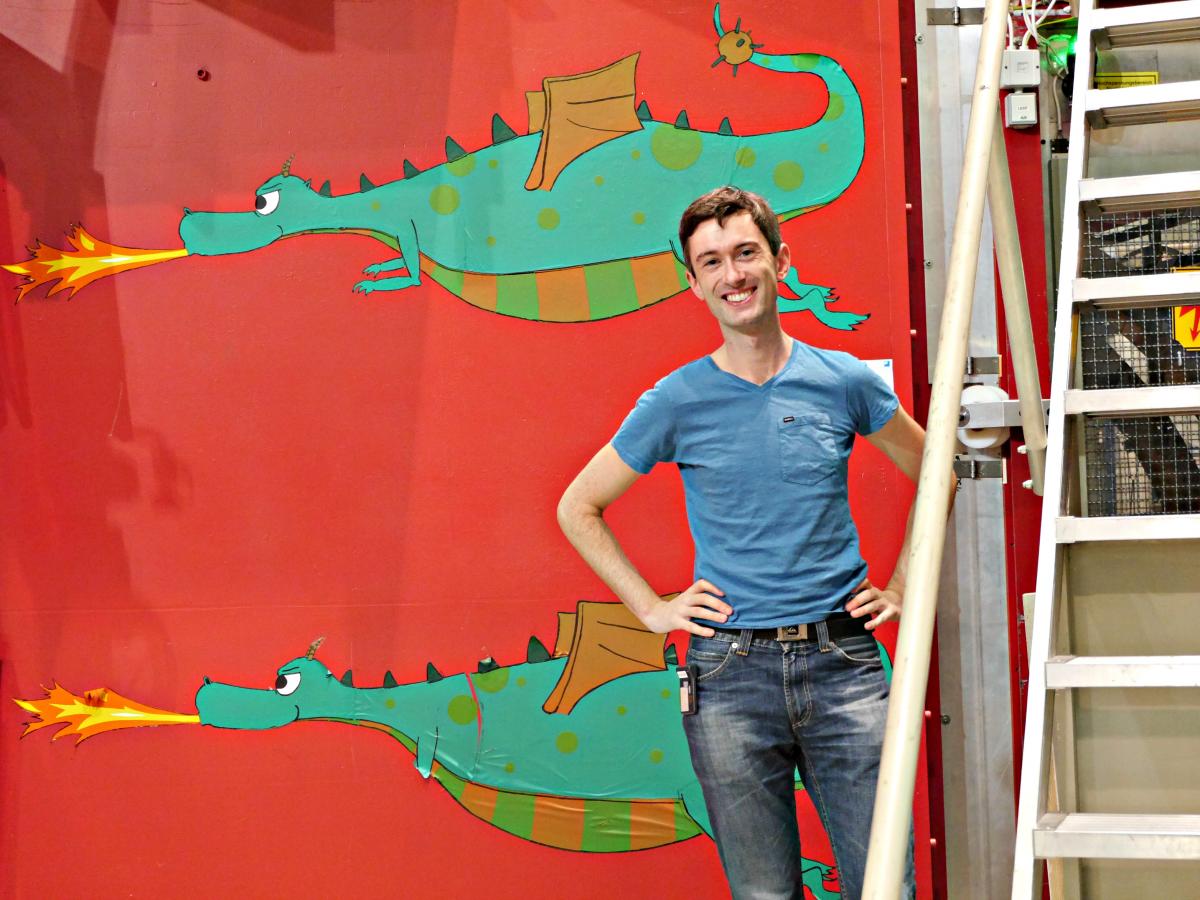
Image courtesy of EUROfusion
Mike Dunne’s decision to take up fusion research was rather accidental. Having completed a bachelor’s degree in astrophysics and physics, Mike was on the hunt for suitable PhD options when his professor informed him about a position in plasma physics. “Since I was planning on studying some kind of fluid or plasma physics anyway, it wasn’t such a big jump,” says Mike, who went on to obtain his PhD before taking up his current position as a EUROfusion fellow at the Max Planck Institute for Plasma Physics in Garching, Germany.
For Mike, now is perhaps the most exciting time to be involved in fusion research. “You’re right on the edge of everything … the subject is growing rapidly around you as ITER [the next-generation fusion experiment] is being built, as plans for demonstration reactors are being made, and [it is exciting] knowing that you’re right in the middle of it, trying to figure out some of the key issues and understand what’s going on.”
Being part of a potential game-changer in the energy landscape is exciting in itself, but for a physicist, fusion research is truly fascinating. Mike works on what is known as boundary physics: what happens at the plasma edge in a fusion experiment. “There are two separate parts here,” he explains, “one of which is inside the confined plasma, and one which is outside. The inside layer is quite narrow, only 2 cm over a 50 cm plasma radius, but separates a tremendous amount of heat from the inside, where fusion will happen, and the colder outside, where the machine walls are. The layer outside of the confined plasma is where particles and heat are ‘exhausted’ from the plasma and pumped away.”
The heat within the inner layer is directly proportional to the fusion power that can be extracted from an eventual power plant. “What I’ve been working on lately is how these two [layers] interact with each other,” he adds. Understanding this interaction will influence the design and operational conditions of future fusion power plants.
Because of growing anticipation and excitement about fusion power and technology, Mike says that the fusion community in Europe has become vibrant and integrated. “There are more opportunities for communication, for discussing your results and ideas with other people,” he explains. “Science is based on collaboration and communication so, apart from data analysis, setting up experiments and programming, reading other people’s papers, listening to talks and lectures, preparing posters and talks for conferences are all part of a typical day,” he says. “All that and coffee… coffee is definitely involved.”
EUROfusion
The European Consortium for the Development of Fusion Energy (EUROfusionw1) comprises representations of 28 European member states as well as Switzerland, and manages fusion research activities on behalf of Euratom. More than 40 European fusion laboratories collectively use the Joint European Torus (JET), which has remained the world’s largest magnetic fusion device since it was built in 1983. Smaller national experiments in Germany, Switzerland and the United Kingdom complement the experimental programme. The aim is to realise fusion electricity by 2050.
Web References
- w1 – To learn more about Eurofusion visit https://www.euro-fusion.org/. For a list of EUROfusion articles in Science in School, see www.scienceinschool.org/EUROfusion