Structural colour: peacocks, Romans and Robert Hooke Understand article
For thousands of years, nature has produced brilliant visual effects. What is the physical principle behind it and how can we use it?
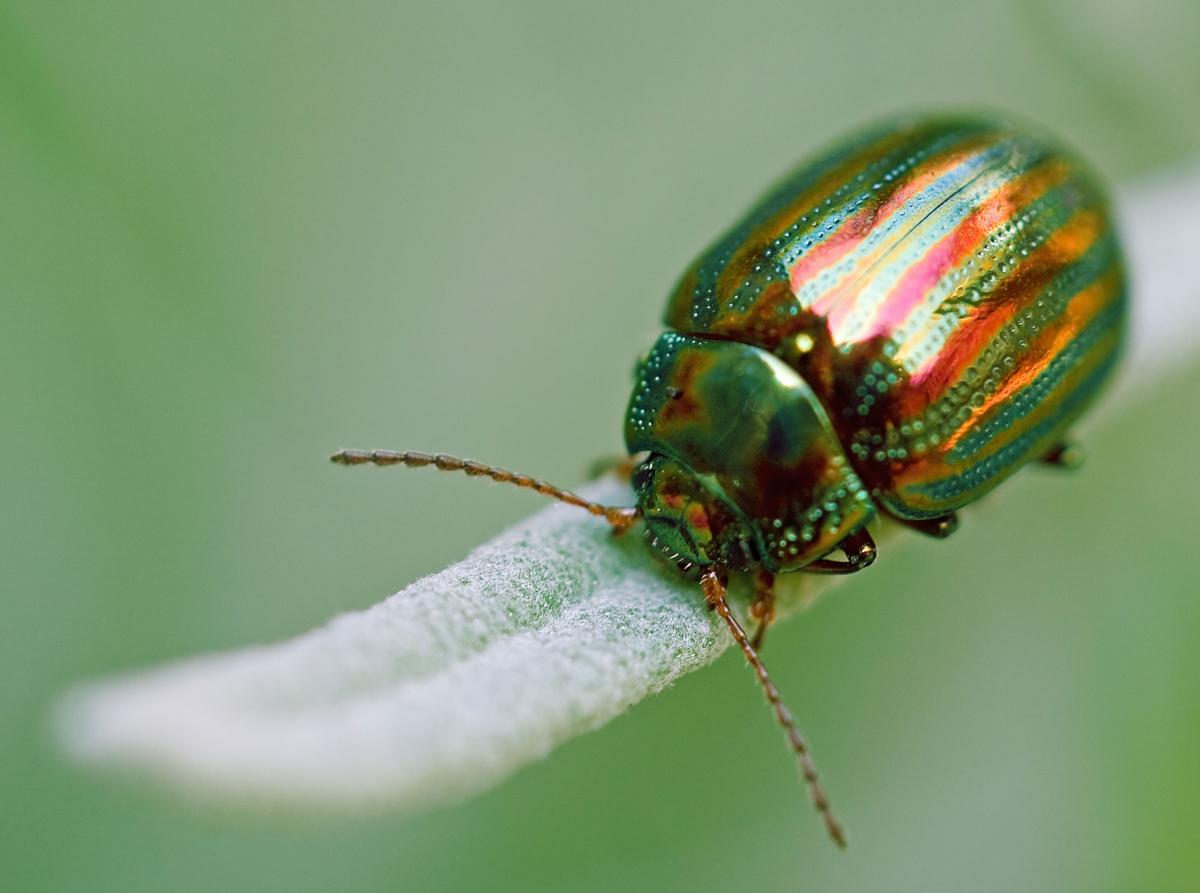
wingcases shells are
responsible of their bright
iridescent colours.
Image courtesy of Matthew
Kirkland; image source: Flickr
Colour is how we visualise different wavelengths of light. The colours we see depend on the light that is reflected or transmitted from objects into our eyes. The most common source of colour is pigmentation: almost everything we see, including our clothes and ourselves, is coloured by pigment molecules.
But there is another way to make colour – one that fruits, beetles, butterflies and peacocks, for example, all use: structural colour. Their colours change depending on your viewpoint, due to microscopic structures on their surfaces that interfere with the reflection of light. Humans have been using structural colour long before knowing its cause, and today scientists are taking inspiration from nature to develop structural colour further. “We try to create materials that manipulate light using the same physical principles as those found in nature,” says Pete Vukusic, a professor of biophotonics at the University of Exeter, UK. “The aim is not to mask or hide things, but to give materials and objects interesting and functional optical properties such as brightness, directionality or iridescence.”
A brief history of wave interference
One sunny afternoon in the 17th century, Robert Hooke discovered something that has gone down in history. Wondering why the colour of peacock feathers changed if viewed from different angles (called iridescence), he plunged a feather into water and made an astonishing discovery: the colour disappeared. “Fantastical”, he wrote. Using a microscope, Hooke saw that the peacock feather was covered in tiny ridges. He concluded that this was evidence that light reflection and refraction were behind colour.
The dual nature of light
Hooke’s explanation of light was soon rejected by Isaac Newton, who thought that light was made of particles (he called them “corpuscles”). Decades later Thomas Young showed that light behaves as a wave by using interference, the phenomenon by which two waves superimpose to result in a wave of bigger or smaller amplitude. In Young’s famous double-slit experiment, light coming from a single source passed through two slits in a solid screen and was observed on a wall behind the screen. The light that came out of these two slits began to show a pattern of light and dark areas, much as ripples propagate in a pond, and if these patterns overlapped the patches became stronger as the waves interfered. This is behaviour that could be explained only if two waves had emerged, one per slit, and then interfered with each other to combine or to cancel out at certain positions.
Later research, however, seemed to contradict Young. The gold leaf experiment showed that electrons are ejected from a metal surface when exposed to electromagnetic radiation, but only if the light has a high enough frequency, and hence energy while the intensity of the source does not determine whether the plate discharges or not. This was evidence that the beams of light and ultraviolet are made up of individual units called photons.
Today we say both that light travels as a wave, but that it also consists of energy packets called photons. Light has a dual nature.
We know now that the colours of many birds and insects arise from structural colour, produced by light interacting with regular structures just a few hundred nanometres in size. These structures break down incident light into several reflected waves that interfere with each other, destroying or reinforcing different wavelengths in different directions and appearing to our eyes as brightly coloured or iridescent. In pigmentary systems the physics is completely different, which explains why structural colour can be much brighter. “Pigments both reflect and absorb light. These absorptive processes, which are intrinsic to the production of pigmentary colour, are the very things which can limit the colour brightness,” says Vukusic.
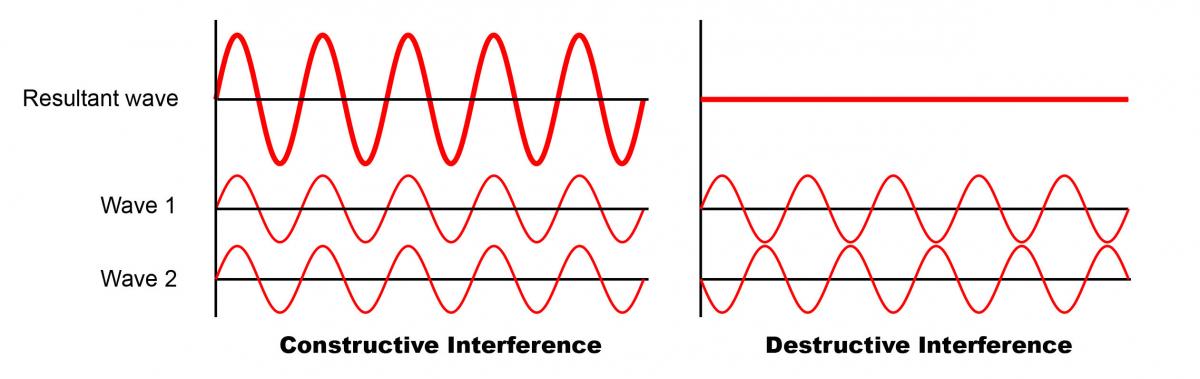
Modified from “Interference of two waves” by Haade (Wikimedia Commons)
Structural colour – past, present and future
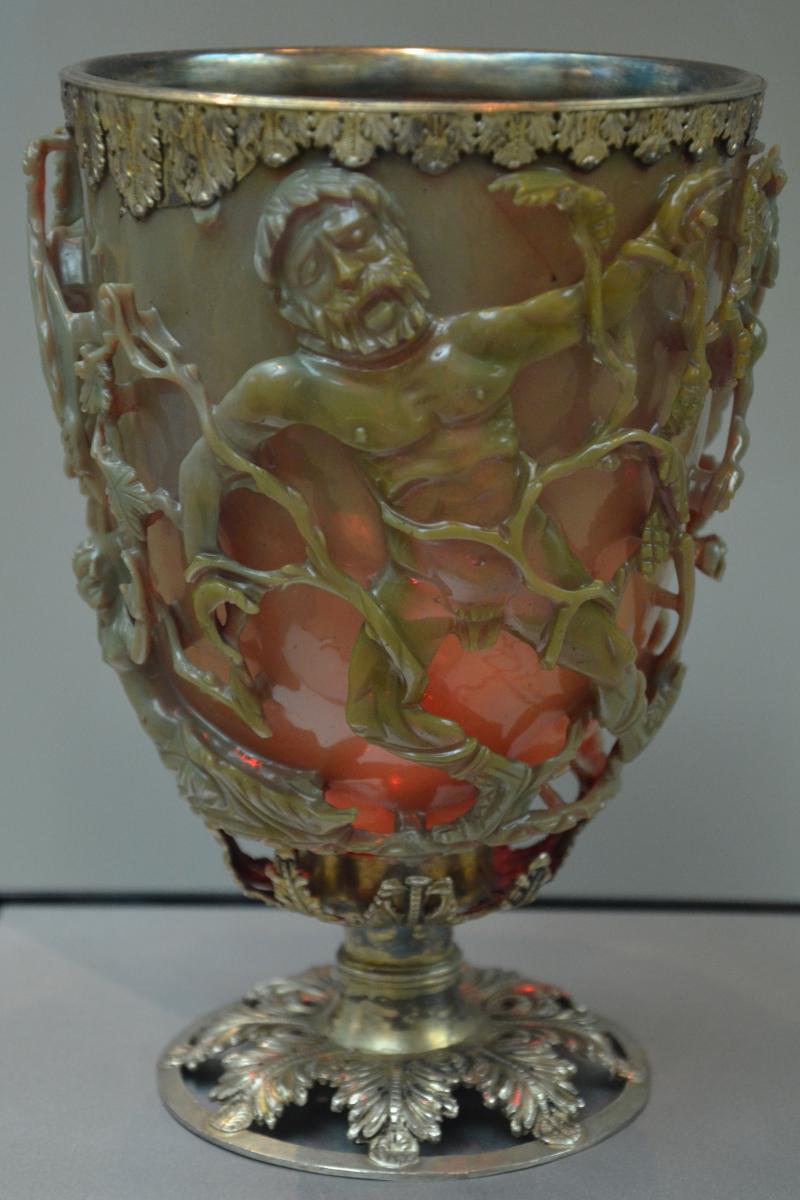
colours of the Lycurgus cup:
reflected light appears green
while transmitted light
shines red.
Image courtesy of Following
Hadrian; image source: Flickr
Over thousands of years of evolution, living organisms have mastered how to manipulate and channel light using delicate, periodically arranged nanostructures. Peacocks, for example, use the brilliant structural colours of their feathers as part of their courtships. Today these biological designs inspire engineers seeking to control light in optical technologies. Scientists are working to produce colour-reflective screens, for e-books or electronic paper, that don’t need their own light to be readable. These displays would use much less power than backlit versions in computers, smartphones and TVsw1. Humans have also used structural colour without even being aware of it.
The Lycurgus cup is a Roman artefact stored in the British Museum in London, UK. Made of glass, it changes its colour from green to red depending on whether the incident light is reflected by the glass or passes through from behind (Freestone, 2007). Like peacock feathers, the striking colour of the Lycurgus cup is due to structural colour, but caused by much smaller structures only several nanometres across – gold nanoparticles dispersed inside the glass. When the surfaces of the metal nanoparticles interact with light, they retain and direct specific colours of light. The Roman glassmakers found by chance that precious metals added to glass gave impressive colour effects, but they could not reproduce it, and the few similar pieces that remain today were all made around the fourth century BC.
Today, at last, humans can use the science of structural colour to make many objects, even the lipstick in your handbag. By using particles that are made of many thin layers and immersing thousands of them in the formulation we get an iridescent lipstick. “The regular nanostructures contained in these particles strongly reflect colours, giving different dramatic and selective appearances in different directions,” says Vukusic, who has also worked with cosmetics firm L’Oréal.
Researchers are also trying to use the physics of light channelling and structural colour in the plastics industry. This is the idea behind Plast4Futurew2, a research project led by Anders Kristensen of the Technical University of Denmark, which is focused on making more environmentally friendly coloured plastics. “With structural colour, you can make plastics using fewer materials and it also facilitates recycling according to a cradle-to-cradle production philosophy,” says Kristensen, referring to the approach that models human industry on nature’s processes, ensuring that energy and material resources flow between compartments without running out or accumulating waste.
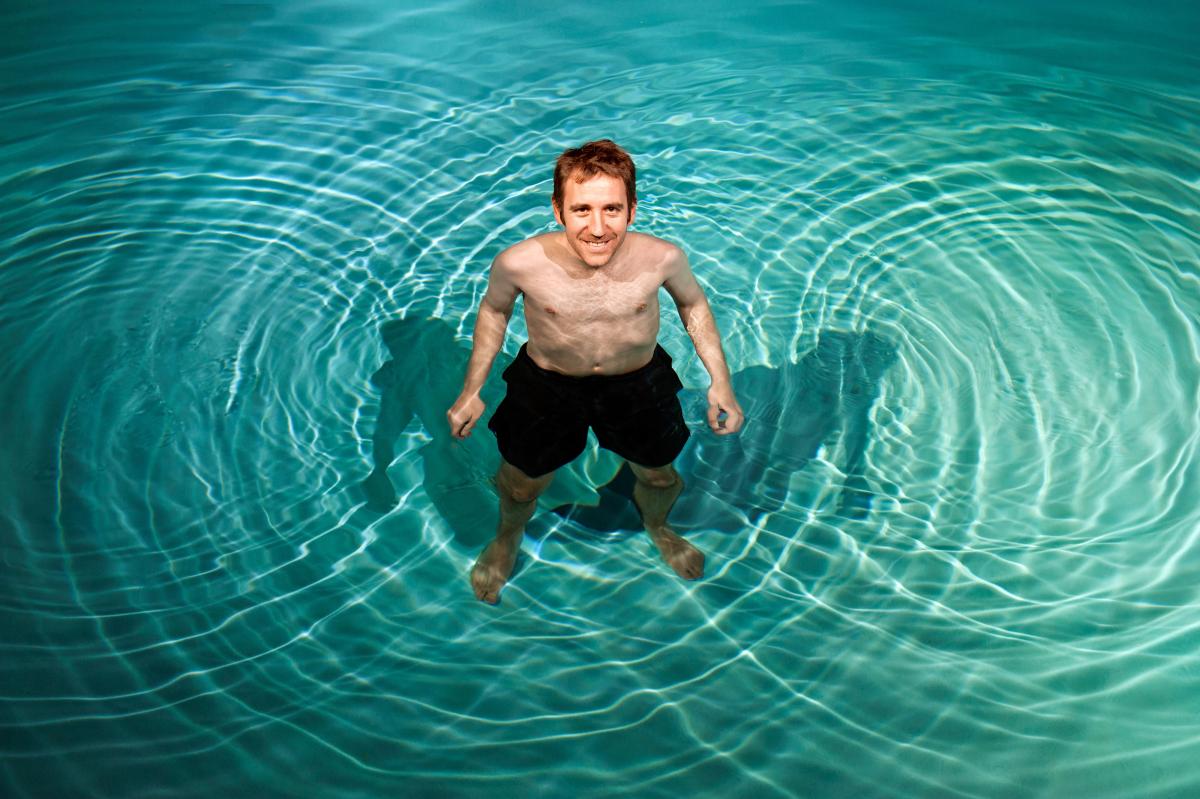
swimming pool. Beat the
water to create two waves
and wait until they meet. In
some areas, the waves
disappear (the water
becomes flat), in others the
waves become bigger.
Image courtesy of ESO/M. Alexander; image source:
Wikipedia
The same physical properties that coloured the Lycurgus glass may someday colour commercially available plastics. Professor Kristensen developed a way to do this just last year. First, his team made a silicon mould with an array of thousands of nanoholes. Then they moulded the plastic and deposited a thin film of aluminium on top. The result was a coloured plastic without pigments, and the colour could be tuned by changing the diameter of the nanoholes (Clausen, 2014). “For recycling, the aluminium can be removed from the plastic, which can then be melted and remoulded, generating a plastic with the same or another colour,” explains Kristensen. “With the traditional system, however, pigments cannot be removed so the recycled plastic will have the same colour,” which means that plastic recycling at the moment is more wasteful and complex.
The Plast4Future project also explores how to give plastics other features such as antifogging or even hydrophobicity (repelling water). All these innovations are of interest to companies that make things from cars to toys: Fiat and LEGO are both active partners in this project.
Far from a curiosity, structural colour is a tool to achieve a cleaner, less polluted world. Such a scenario could hardly be imagined by the major historical figures in this story, but they all helped us to understand what we know today about this extraordinary source of visual effects.
More about European XFEL
European XFEL is a facility that is under construction in the Hamburg area of Germany that will be able to study basic properties of matter for various disciplines, including nanotechnology, using ultrabright flashes of X-ray laser light. Since the X-ray laser can make visible structures at the atomic scale and at timescales near a quadrillionth of a second, it will be able to study the details of interactions of nanoparticles with other matter and with light. This could open the door for a better understanding of how nanoparticles are structured, how they behave in terms of reactivity and atomic motion, and why they function in certain ways in different environments such as solutions or aerosols.
References
- Clausen JS et al (2014) Plasmonic Metasurfaces for Coloration of Plastic Consumer Products. Nano Letters 14: 4499–4504. doi: 10.1021/nl5014986
- Freestone I et al (2007) The Lycurgus Cup: A Roman nanotechnology. Gold Bulletin 40: 270–277. doi: 10.1007/BF03215599
Web References
- w1 – Read more about how next-generation e-readers can use structural colour to improve their high-resolution colour displays.
- w2 – Visit the Plast4Future website.
Review
Structural colour and its scientific application are likely to be of great interest to pupils. This article is very interesting and gives a good overview of optical effects and how colour is ‘produced’.
The article tries to stimulate questions like:
- How is our eye able to see colours physically?
- What is colour? Give a physical explanation.
- How can we create colours?
- Explain the dual nature of light.
- How are colours used in nature and in technology?
- What is structural colour?
- Give some examples of the use of structural colour. How do these items create colour?
- Explain some technical applications of structural colour.
Gerdt Vogt, Higher Secondary School for Environment and Economics, Yspertal, Austria