More than meets the eye: unravelling the cosmos at the highest energies Understand article
Claudia Mignone and Rebecca Barnes explore X-rays and gamma rays and investigate the ingenious techniques used by the European Space Agency to observe the cosmos at these wavelengths.
Viewed with the naked eye, binoculars or a telescope, the starry night sky is an overwhelming and tranquil sight. But if we could view the sky in highly energetic X-rays and gamma rays, rather than the visible light perceived by our eyes, we would see a very different picture – a dramatic cosmic light showw1 (Figure 1).
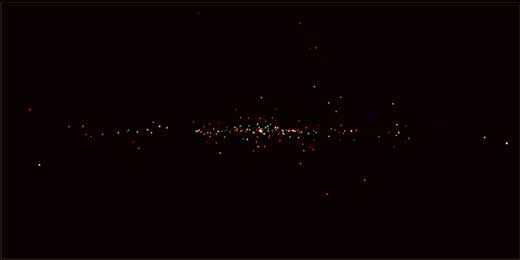
Images courtesy of ESA / F Lebrun / CEA Saclay, Service d’Astrophysique (above); ESO / S Brunier (below)
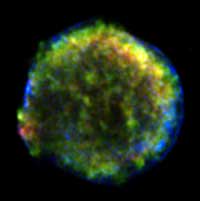
supernova remnant as
viewed by ESA’s XMM-
Newton. This remnant is
relatively young and is
associated with a supernova
explosion that was observed
in 1572 by the Danish
astronomer Tycho Brahe.
Click on image to enlarge
Image courtesy of Marco
Iacobelli (XMM-Newton SOC)
and ESA
Some of the most powerful and violent phenomena in the Universe shine brightly at these short wavelengths, such as supernova explosions – the fiery demise of a massive star’s life – and black holes, rapidly devouring matter. As a sign of their dynamic nature, many sources of X-rays and gamma rays exhibit distinct changes in their brightness, even over very short periods of time. Gamma-ray bursts, for example, appear as sudden bright flashes that last just a few seconds. These bursts arise from possibly the most extreme explosions in the cosmos (to learn more, see Boffin, 2007). Furthermore, X-rays and gamma rays are released through different physical processes than those responsible for the emission of visible light. This means that galaxies and other astronomical objects look different when imaged at the high-energy end of the electromagnetic (EM) spectrumw2 (Figures 2 and 3).
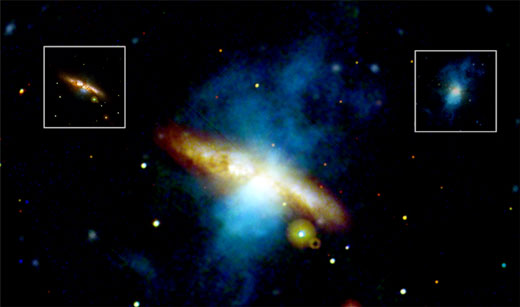
Image courtesy of ESA
This revolutionary view of the cosmos was revealed to astronomers in the early 1960s, with the beginning of the space age, when rockets and satellites allowed specially developed instruments to be carried beyond the obscuring barrier of Earth’s atmospherew3. The European Space Agency (ESA; see box)w4 soon joined in, with the gamma-ray mission COS-B (1975) and the X-ray observatory EXOSAT (1983). Today, ESA operates two such observatories: the X-ray Multi-Mirror satellite (XMM-Newton), launched in 1999, and the International Gamma-Ray Astrophysics Laboratory (INTEGRAL), launched in 2002.
How do they work? As we explained in an earlier article (Mignone & Barnes, 2011), there is no physical distinction between X-rays, gamma rays, visible light and other types of EM radiation. All are forms of light, differing only in their wavelength (or, as the three are correlated, their frequency or energy; Figure 4). However, depending on their wavelength (or frequency, or energy), they interact very differently with matter. This has major implications for astronomy.
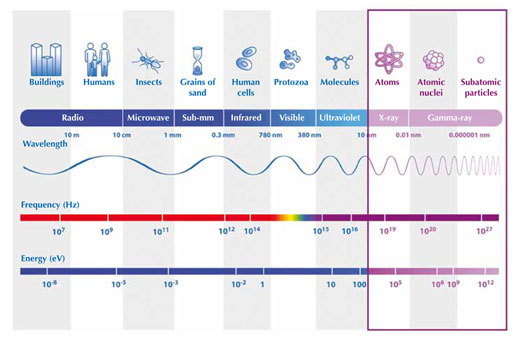
Image courtesy of ESA / AOES Medialab
Traditional optical systems, such as our eyes, cameras, microscopes or telescopes, rely on lenses (or mirrors) that refract (or reflect) light rays and focus them into a single point to produce images. However, this is difficult with some light rays. Because X-rays and gamma rays have wavelengths of a similar size to atoms and sub-atomic particles, respectively, they cannot easily be reflected or focused like visible light, but tend instead to be absorbed when they strike denser materials (Figure 5).
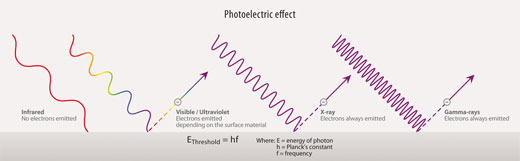
Click on image to enlarge
Image courtesy of ESA / AOES Medialab
The fact that X-rays and gamma rays are absorbed by dense materials makes them suitable for many applications, including medical scans and investigations of materials. For astronomers, however, it is a problem: being easily absorbed, these types of radiation are very difficult or impossible to focus; thus obtaining sharp images of their sources is a challenge.
Nonetheless, scientists have developed techniques to detect X-rays and gamma rays coming from the cosmos. They differ greatly from techniques used in traditional optics and that, together with the fact that they operate in space, means that telescopes for high-energy astronomy look nothing like optical telescopes.
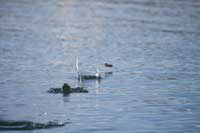
Image courtesy of Killy Ridols;/
image source: Wikimedia
Commons
X-ray observing techniques
Although it is difficult to reflect X-rays, it is not impossible if they hit the telescope’s mirror at a very small angle – think of a pebble skimming across the surface of the water. However, whereas an incidence angle as large as 20° will allow the stones to bounce, X-rays can be reflected only at much smaller angles: 1° or even less. The X-rays must barely graze the mirror, or they are likely to be absorbed.
To achieve this small angle – and focus the X-rays to a single point – the mirrors used in X-ray telescopes look rather like a funnel (Figure 6). In fact, the mirror shape is a combination of a paraboloid and a hyperboloid, ensuring that the X-rays that graze it are reflected twice. In this way, light is focused onto a detector to form an image of the X-ray source.
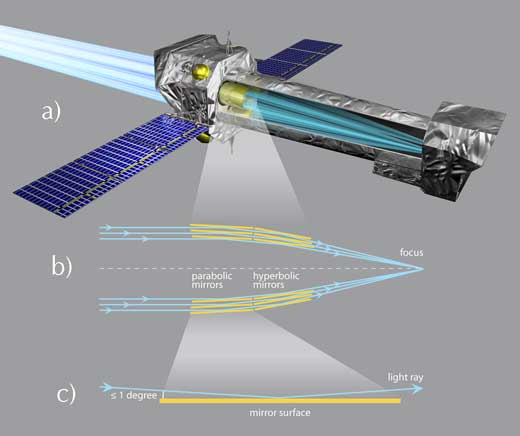
a) The light path of X-rays through XMM-Newton. The spacecraft carries three telescopes each consisting of
58 nested, gold-coated, tube-like mirrors.
b) The combination of parabolic and hyperbolic mirrors used is shown in cross section through one of the telescopes
c) X-rays that graze the mirror surfaces are reflected twice and focused onto a detector. The X-rays must graze the mirror at angles of 1° or even less, or they are likely to be absorbed. Click on image to enlarge
Image courtesy of ESA / AOES Medialab
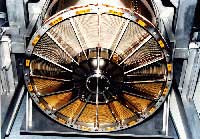
constituting one of the three
telescopes on board
XMM-Newton
Image courtesy of ESA
This ingenious technique, called grazing incidence optics, has one main drawback: to be reflected and focused, the X-rays must be travelling almost parallel to the tube-like mirrors, so these telescopes collect only limited amounts of X-ray radiation. A powerful telescope is one that collects large amounts of light from distant cosmic sources; this is usually achieved with very large mirrors. In contrast, to maximise their power, X-ray telescopes have several mirrors nested within one another, creating a structure that resembles a giant leek. The three telescopes on board ESA’s XMM-Newton space observatory, for example, each consist of 58 nested mirrors (Figure 7)w6.
Besides their bizarre shape, XMM-Newton’s mirrors differ from conventional telescope mirrors in that they are made of gold-coated nickel rather than aluminium-coated glass: the heavier elements are more likely to reflect incoming X-rays (to learn more, see Singh, 2005).
Gamma-ray observing techniques
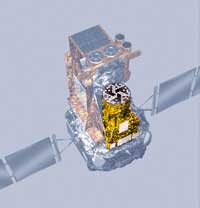
of INTEGRAL highlighting SPI,
one of the coded-mask
instruments on board the
spacecraft.
Click on image to enlarge
Image courtesy of ESA / AOES
Medialab
If focusing X-rays is challenging, focusing gamma rays – the most energetic form of light – is almost impossible. To produce images of cosmic sources in this portion of the EM spectrum, therefore, astronomers had to find alternative methods.
Many instruments for gamma-ray astronomy, including those on board ESA’s INTEGRAL space observatory, rely on a technique called coded-mask imaging. This works similarly to a pinhole camera, which has no lens, just a tiny hole through which light rays pass, projecting an inverted image on the opposite wall of the camera.
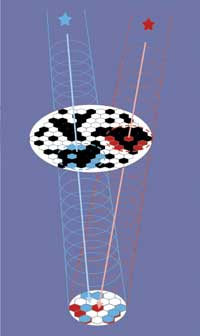
mask camera works: gamma-
rays from two different
astronomical sources pass
through the mask’s holes.
Some of the incident gamma
-rays can pass through the
mask and illuminate pixels
on the detector below (shown
in blue and red, depending
on the source), while others
are blocked by the mask’s
opaque spots, casting
shadows on the detector
(shown in white).
Click on image to enlarge
Image courtesy of ESA / AOES
Medialab
In place of the pinhole camera’s single hole, a coded-mask camera has a mask with a special pattern of holes and opaque spots in front of a detector. Gamma rays that pass through the holes illuminate some pixels on the detector, while others are blocked by the mask’s opaque spots and cast shadows on the detector.
The pattern of bright and dark pixels contains information about the location of gamma-ray sources in the sky, and the intensity of the illuminated pixels gives information about their brightnessw7. Albeit not detailed, the resulting images are useful to probe some of the most powerful phenomena in the Universe (Figuras 8a and 8b, 9 and 10).
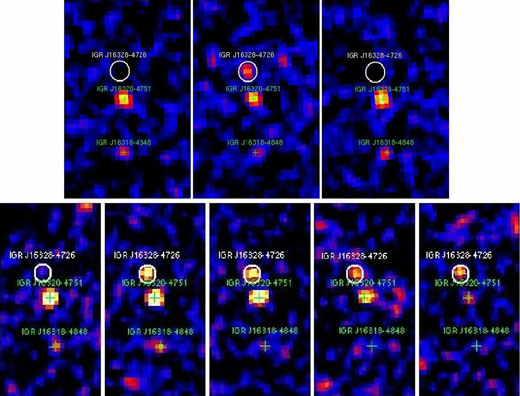
Image courtesy of ESA / INTEGRAL / M Fiocchi
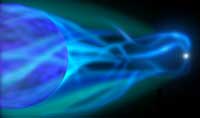
of a supergiant fast X-ray
transient.
Click on image to enlarge
Image courtesy of ESA
Coming up…
As you read this article, ESA’s XMM-Newton and INTEGRAL observatories are circling Earth, keeping watch over the ever-changing, high-energy Universe and helping to unravel celestial wonders. In our next article, we will explore some of these phenomena, such as the turbulent life and death of stars in the Milky Way, and gigantic black holes at the centres of distant galaxies.
More about ESA
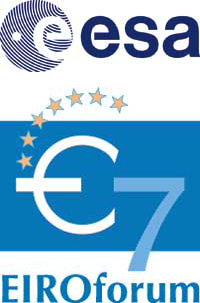
The European Space Agency (ESA)w4 is Europe’s gateway to space, organising programmes to find out more about Earth, its immediate space environment, our Solar System and the Universe, as well as to co-operate in the human exploration of space, develop satellite-based technologies and services, and to promote European industries.
The Directorate of Science and Robotic Exploration is devoted to ESA’s space science programme and to the robotic exploration of the Solar System. In the quest to understand the Universe, the stars and planets and the origins of life itself, ESA space-science satellites peer into the depths of the cosmos and look at the furthest galaxies, study the Sun in unprecedented detail, and explore our planetary neighbours.
ESA is a member of EIROforumw8, the publisher of Science in School.
References
- Bernardelli A (2010) Stage lights: physics and drama. Science in School 17: 41-45.
- Boffin H (2007) Fusion in the Universe: gamma-ray bursts. Science in School 7: 61-63.
- Mignone C & Barnes R (2011) More than meets the eye: the electromagnetic spectrum. Science in School 20: 51-59.
- Singh KP (2005) Techniques in X-ray Astronomy. Resonance – Journal of Science Education. 10(6): 15-23.
Web References
- w1 – For a movie based on INTEGRAL data, comparing the appearance of the sky as observed in visible light and in gamma rays, as well as the variability of the gamma-ray emission of sources in the bulge of the Milky Way, see: http://sci.esa.int/GalacticBulge_video
- w2 – To watch an animation showing the different appearance of the galaxy M 82 in visible light, ultraviolet and X-rays, see: http://sci.esa.int/science-e-media/img/40/M82Zoom410x354.gif
- w3 – To read or listen to Riccardo Giacconi’s 2002 Nobel lecture, ‘The Dawn of X-ray Astronomy’, visit http://nobelprize.org/nobel_prizes/physics/laureates/2002/giacconi-lecture.html
- w4 – For more information about ESA, see: www.esa.int
- To learn more about the activities of ESA’s Directorate of Science and Robotic Exploration, visit: http://sci.esa.int
- Education materials produced by ESA are freely available to teachers in the 18 ESA member states. Many are translated into several European languages. See: http://www.esa.int/Education/Teachers_Corner
- Instructions and patterns for building paper models of many ESA spacecraft (including XMM-Newton and INTEGRAL) can be downloaded here: https://www.esa.int/Education/Classroom_tools
- The ESA multimedia gallery offers over 10 000 images, videos and animations related to space. See: www.esa.int/esa-mmg/mmghome.pl
- w5 – For an interactive simulation of the photoelectric effect, as well as some associated activities, see the PhET website (http://phet.colorado.edu) or use the direct link: http://tinyurl.com/679wytg
- To learn more about the photoelectric effect, see: http://physics.info/photoelectric
- w6 – For an animation of the light path through XMM-Newton’s telescopes, see: http://sci.esa.int/jump.cfm?oid=45618
- w7 – To learn more about the coded-mask camera, see www.sron.nl/~jeanz/cai/coded_intr.html
- w8 – To find out more about EIROforum, visit: www.eiroforum.org
Resources
- The Science@ESA vodcasts explore our Universe through the eyes of ESA’s fleet of science spacecraft. Episode 5 (‘The untamed, violent Universe’) offers a glimpse of the hot, energetic and often violent universe, and the ESA missions that detect it using X-ray and gamma-ray astronomy. See: http://sci.esa.int/vodcast
Institutions
Review
This article explains simply and comprehensibly how X-rays and gamma rays are collected from cosmic sources using modern space telescopes, and it provides some dramatic images.
For science teachers in primary schools, the article may provide motivation to build a model telescope in lessons, for example using recycled materials – or to use the downloadable satellite models on the ESA websitew4. The colourful images can also form part of a class exhibit.
Science or physics teachers at secondary school (students aged 11-16) can link to the topic of gamma-ray imaging techniques using a pinhole camera. This would be appropriate in optics lessons, emphasising that both the pinhole camera and coded-mask imaging work without an optical lens.
Images taken by ESA’s observatoriesw4 would be a useful support for teaching space observation, helping to familiarise students with the different astronomical phenomena (e.g. galaxies, black holes, supernovas, neutron stars, or the annihilation of matter and anti-matter) mentioned in the article. It could also encourage students to do some research of their own on related areas within the curriculum.
For teachers of older school students, it would be interesting to discuss the type of telescopes for high-energy astronomy that are on board the space observatories XMM-Newton and INTEGRAL, and the techniques used to filter the data until the images are fully extracted (this could be linked to IT lessons). Students could compare the structure of telescopes in the high-energy end of the spectrum to that of the optical telescope, and investigate the difficulties encountered when building them.
Stephanie Maggi-Pulis, Malta