Solar energy: silicon solar cells Understand article
With oil reserves running out, silicon solar cells offer an alternative source of energy. How do they work and how can we exploit their full potential?
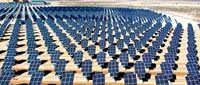
land on Nellis Air Force Base,
Nevada, USA, this solar
photovoltaic array will
generate 15 MW of solar
power for the base
Image courtesy of US Air Force
photo / Airman 1st Class
Nadine Y Barclay; image
source: Wikimedia Commons
Indirectly, the Sun is the source of most of the energy we use on Earth: not only of fossil fuels and biomass, but also wind and tidal energy, to mention just a few. Increasingly, there is interest in capturing the energy from the Sun more directly, using photovoltaic cells.
A relatively old, medium-sized star made of hot plasma, the Sun radiates energy as electromagnetic radiation over a wide spectrum. At a distance of 150 million kilometres, our planet receives an irradiance of around 1366 W/m2 (1 W= 1 J·s) from the Sun, but not all of this actually reaches us because Earth’s atmosphere reflects and absorbs about 30 % of this energy. Nonetheless, every square metre of Earth’s surface receives an average of nearly 1000 Joules per second from the Sun.
To put this into perspective, the total energy consumed globally in 2010 was around 5 x 1020 J. If we assume that our planet is a perfect sphere with a radius of 6370 km, Earth receives 1.8 x 1017 J/s, of which about 1.3 x 1017 J/s reaches Earth’s surface. Thus in one hour, the Sun provides Earth with all the energy we need for a whole year.
It isn’t quite that simple, however. Due to meteorological factors, the Sun’s declination and Earth’s rotation, the irradiance is actually closer to 230 W/m2. If we repeat the last calculation using that figure, the time needed to power Earth with energy from the Sun for a year is about five and a half hours – still an impressively short time.
Solar radiation is therefore a promising energy reservoir, but how can we collect it and use it?
What happens inside a photovoltaic cell?
The foundations for modern solar energy collection were laid in 1839, when the French physicist Edmond Becquerel observed an increase in the electrical conductivity of some materials when they were exposed to light; this became known as the photovoltaic effect. It was not until quantum mechanics was developed, though, that the phenomenon was explained. Electromagnetic radiation can be described as a stream of quantum objects called photons. When these photons are absorbed by some materials, they can promote electrons in the material into a higher energy state (the conduction band), potentially enhancing the material’s conductivity.
Semiconductors, such as silicon, are photovoltaic because a photon’s energy matches that required to move one of the semiconductor’s electrons up into the conduction band. However, semiconductors themselves have few free electrons and, therefore, low conductivity. To increase their electrical conductivity, tiny amounts of other materials (impurities) can be added, a process called doping.
Doped silicon is the most frequently used material in electronics. Pure silicon has four valence electrons that it shares with four neighbouring atoms. Adding impurities with more or fewer valence electrons (such as phosphorus or boron) modifies the conductivity properties of the material. Phosphorus has five valence electrons and, when a phosphorus atom is surrounded by silicon atoms, the fifth electron is only loosely bound. This means it can easily reach the conduction band, helping to increase the material’s conductivity. Phosphorus-doped silicon is called n-type (negative type) since doping increases the number of negative free charges (electrons). In contrast, boron has only three valence electrons, and the lack of one electron in the silicon lattice creates a ‘hole’. As free electrons move through the lattice, from one hole to another, the positively charged holes appear to move through the material. Boron-doped silicon is known as p-type (positive-type) silicon.
These phenomena can be exploited in solar cells to collect energy from the Sun and transform it into electrical energy. The simplest solar cell is formed by the junction of two semiconductors, one p-doped and one n-doped, called a p-n junction. At this junction, the electrons in the n-type silicon ‘see’ the holes in the p-type silicon and travel to fill them – creating electron-hole pairs. When a photon strikes one of these pairs, however, it breaks apart and the flow through the material of these newly freed charge carriers, both positive and negative, generates an electric current.
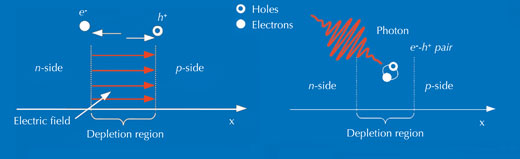
Image courtesy of Enrique García-García
Not all the freed charge carriers generated by this process will contribute to the current, however. Instead, a significant proportion of the electrons and holes will pair up again, generating heat. This reduces the energy conversion efficiency of a photovoltaic material: the percentage of the incoming solar energy that is converted into electrical energy. This is one of the most important parameters of a solar cell’s quality. Currently, commercially available silicon solar cells are approximately 20 % efficient, but extensive efforts are being made to improve this value.
Photovoltaics in practice
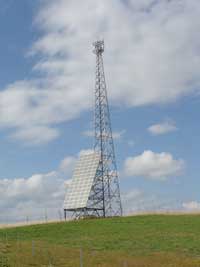
powered by photovoltaics
Image courtesy of
Aomorikuma; image source:
Wikimedia Commons
We now know what is happening inside a solar cell, but what are the practicalities of using solar cells to capture energy from the Sun? A standard solar module is approximately 1.3 m2 and consists of an array of around 50 single solar cells. Depending on the technology, one module will deliver about 200 W, so an assembly of five modules can supply the energy needs of an average household – about 1 kW. In theory, the total energy demand of Europe could be satisfied by covering just 1 % of the continent with solar cells. Realistically, however, solar power is only going to be part of the solution to our energy needs.
In Europe in 2010, about 1 % of energy was obtained using photovoltaic technology, but optimistic estimates of what proportion of Europe’s energy needs could, realistically, be met by solar power range from 30 to 50 %. More precise figures are not yet possible because the necessary technological innovations are still being developed.
One of the limitations of solar energy is that the amount of electricity generated by solar cells is strongly dependent on environmental factors including cloudy weather; the angle at which the sunlight strikes the panel; snow, rain, leaves and other debris on the surface; and, of course, night time. One way to address these issues is by incorporating solar energy into a smart grid, a new concept of a power grid that co-ordinates electricity production from several sources – including solar cells, thermal generators and nuclear plants – to meet consumer demand. In this kind of power distribution, solar cells are playing an increasingly important role.
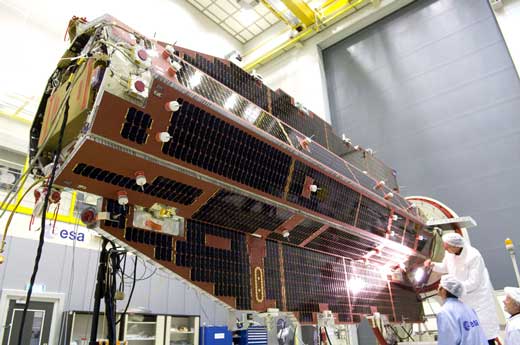
Image courtesy of ESA
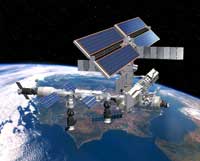
International Space Station,
which has solar panels
comparable in span to the
size of a football pitch,
generating an impressive 92
kW of power
Image courtesy of ESA / D
Ducros
They are also becoming more popular on a smaller scale where the electricity produced may be used on-site – in people’s houses, for roadside telephones, at industrial plants, and on boats, cars and even the International Space Stationw1.
So while we are still unreachably far from meeting our annual global energy needs from five hours of sunshine, photovoltaic technology is increasingly a feasible source of energy. Next time you switch on the kettle or the television, think of the sunlight that helped to power it.
Organic photovoltaics
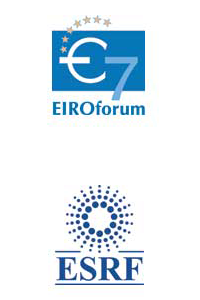
Photovoltaic panels based on crystalline semiconductors – as described in this article – are relatively expensive to produce and process. An alternative is offered by organic photovoltaic materials, which allow large solar panels on flexible substrates to be produced using low-cost processes such as inkjet printing. However, much more research is necessary to improve their efficiency.
Most organic photovoltaic devices are based on thin films comprising an electron-accepting component (such as a fullerene derivative) and an electron-donating component (usually a conjugated polymer) between two electrodes. An important requirement is to mix these two components to obtain a continuous network of donor and acceptor paths for the carriers (electrons or holes) to reach the appropriate electrode (see image). Analysis with synchrotron X-rays at the European Synchrotron Radiation Facility (ESRF) is a good way to examine these materials closely, enabling their characteristics to be improved.
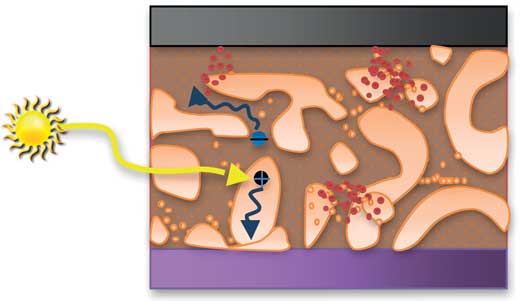
Image courtesy of ESRF
To learn more, see the ESRF websitew2.
ESRF is a member of EIROforumw3, the publisher of Science in School.
Web References
- w1 – To learn more about how solar energy is used to power space missions, download the European Space Agency’s booklet Satellite Power Systems: Solar Energy Used in Space.
- w2 – Situated in Grenoble, France, ESRF operates the most powerful synchrotron radiation source in Europe.
- Find out more about organic photovoltaics at ESRF.
- w3 – EIROforum is a collaboration between eight of Europe’s largest inter-governmental scientific research organisations, which combine their resources, facilities and expertise to support European science in reaching its full potential. As part of its education and outreach activities, EIROforum publishes Science in School.
Resources
- To learn more about how the Sun’s energy is absorbed by Earth’s atmosphere, see:
- Shallcross D, Harrison T (2008) Climate change modelling in the classroom. Science in School 9: 28-33.
- To learn how to build your own solar cell, see:
- Shallcross D, Harrison T, Henshaw S, Sellou L (2009) Looking to the heavens: climate change experiments. Science in School 12: 34-39.
- Tatalovic M (2010) Solar cars: the future of road transport? Science in School 16: 50-53.
- The European Photovoltaic Industry Association’s website has a list of frequently asked questions and answers concerning photovoltaic materials.
- The University of New South Wales, Australia, has produced an electronic book on photovoltaic materials.
- The website of the US Department of Energy’s National Renewable Energy Laboratory provides information on photovoltaics and solar energy.
- The EU-MENA project is investigating using deserts in the Middle East and North Africa to generate solar energy for the region, and also for Europe.
Review
The harnessing of solar energy is a topic that is currently widespread amongst various countries worldwide, especially in view of the rising awareness of climate change and the depletion of non-renewable sources of energy. Countries are becoming more conscious of the need to reduce their reliance on non-renewable sources of energy and, at the same time, to adopt policies favouring renewable sources of energy.
This article provides a very good example of how semiconductors are used in photovoltaic cells and gives a general idea of the energy radiated by the Sun and how much of it is actually collected through solar devices. The article’s main concepts can be included as part of broader topics such as photoelectric effect, conductors, insulators, intrinsic and extrinsic semiconductors, energy band theory and electric current.
Comprehension and extension questions could include:
- The Sun is a very powerful source of renewable energy. Why is it then that the energy generated from solar energy is just a small percentage of what we actually consume annually?
- Explain why semiconductors such as silicon are used in photovoltaic cells.
- The conductivity of a semiconductor can be increased by doping the material. Explain how this process produces p-type and n-type semiconductors.
- How are these doped semiconductors used in photovoltaic cells to capture solar energy?
- How efficient are these photovoltaic cells and in practice, how much of Europe’s energy is obtained using photovoltaic technology?
- What are the factors that limit the efficiency of solar energy collection?
This article is ideal for linking the topics of renewable energy (such as solar) to environmental issues. The positive impact of using solar photovoltaic cells or solar heaters to generate energy has been amply documented. However, we could also prompt our students to research and reflect on the impact that these devices will have on our planet in future years. Which materials are used to construct solar heaters, solar panels and photovoltaic cells? Is the manufacturing process harmful to the environment in any way? What is the lifespan of this equipment, and how is it disposed of upon reaching its end of life? Are these materials manufactured from non-renewable materials? If yes, how long will the materials last? In view of the efficiency of these devices, are they economically viable to produce and maintain?
Catherine Cutajar, Malta